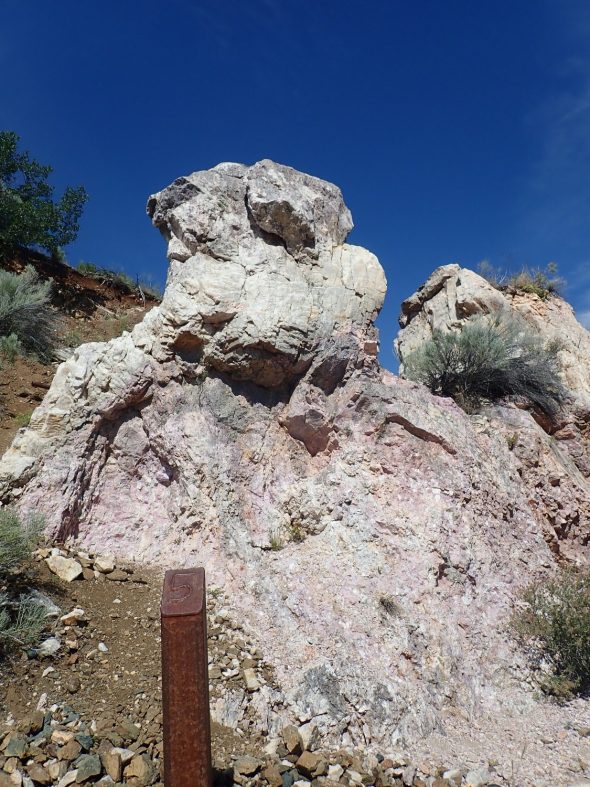
Wanderlusting the Harding Mine, again
I’ve been to the Harding Mine three times before. The first was as a middle-school kid, for a science field trip, which is one of the great happy memories of my childhood. I still have some good specimens from that trip. The second was around nine years ago, with Bruce Rabe, where I picked up a couple more good samples. The last was with the Los Alamos Geological Society just a year ago.
The last trip was not a bad day at all, but I lamented my failure to find any beryl, one of the major ore minerals of the mine. (Of beryllium, if that was not obvious.) Also, I was sufficiently preoccupied with minerals and with my conversation with Gary Stradling that I didn’t really pay much attention to the geologic setting. This trip I would look more at the geology of the mine, perhaps get a specimen or two more if they were good, definitely try to get some beryl, and see if there really is any tantalite placer in the drainages below the mine.
The LAGS meeting point where the mine road leaves the highway.
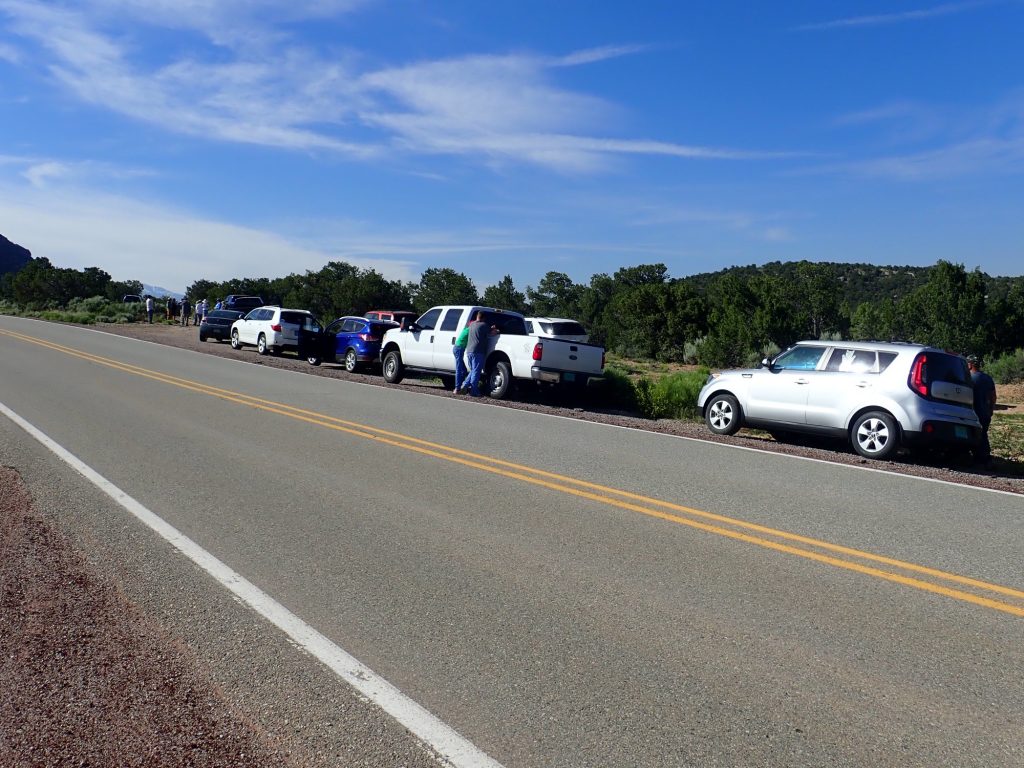
As usual, Patrick Rowe is our field guide, and he gives us a briefing.
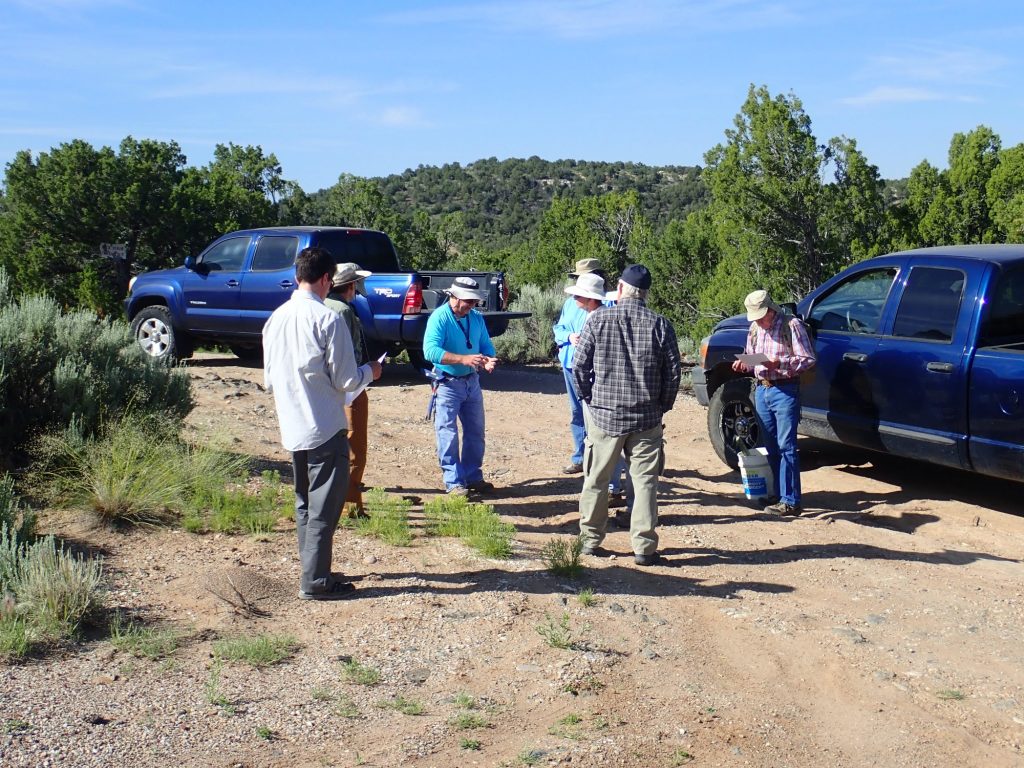
The area here is underlain by a fairly pretty amphibolite, but I failed to get a photograph.
We head up the dirt road to the first parking area. The gate is locked and we will have to hike the rest of the way in.
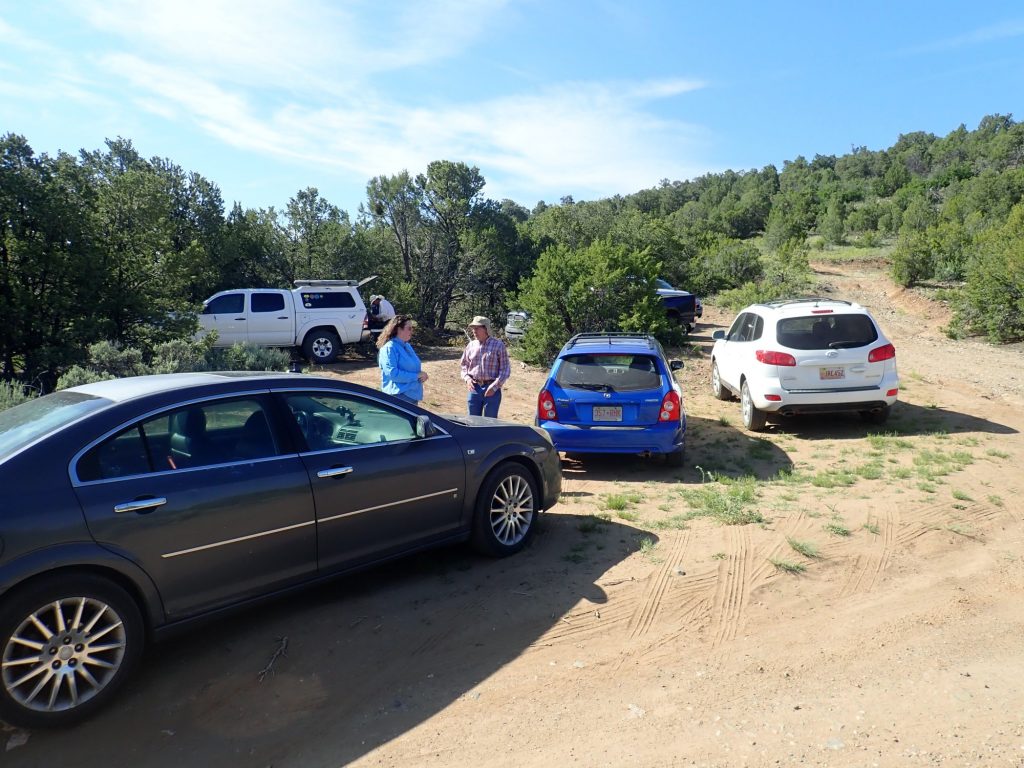
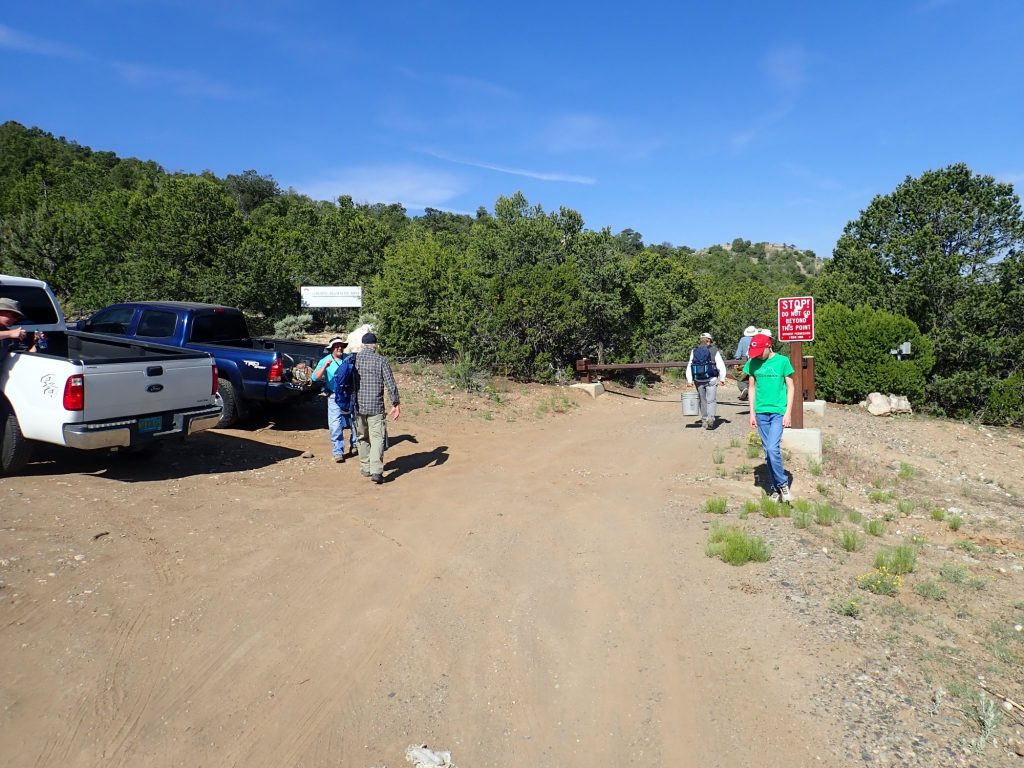
It’s a pleasant walk, except that there seem to be a lot of bugs around. I found that I had not put any DEET in my pack; Patrick Rowe lends a few squirts of his.
Here, north of the mine, the area is underlain by mica schist.
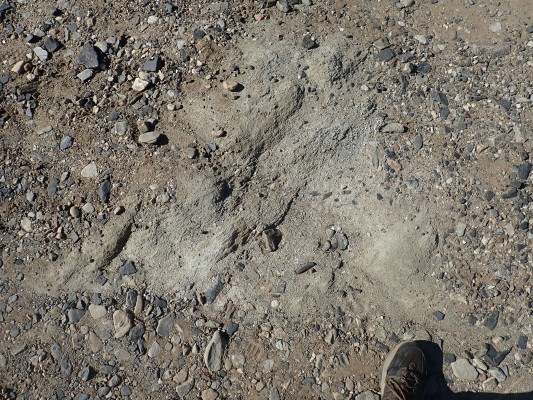
This is mapped on my (somewhat old) geologic map as Vadito Group schist. My guess would be that it belongs to the Rinconada Formation. This is ancient rock by New Mexico standards, around 1.7 billion years old.
A little further on, the road cuts through a small pegmatite dike.
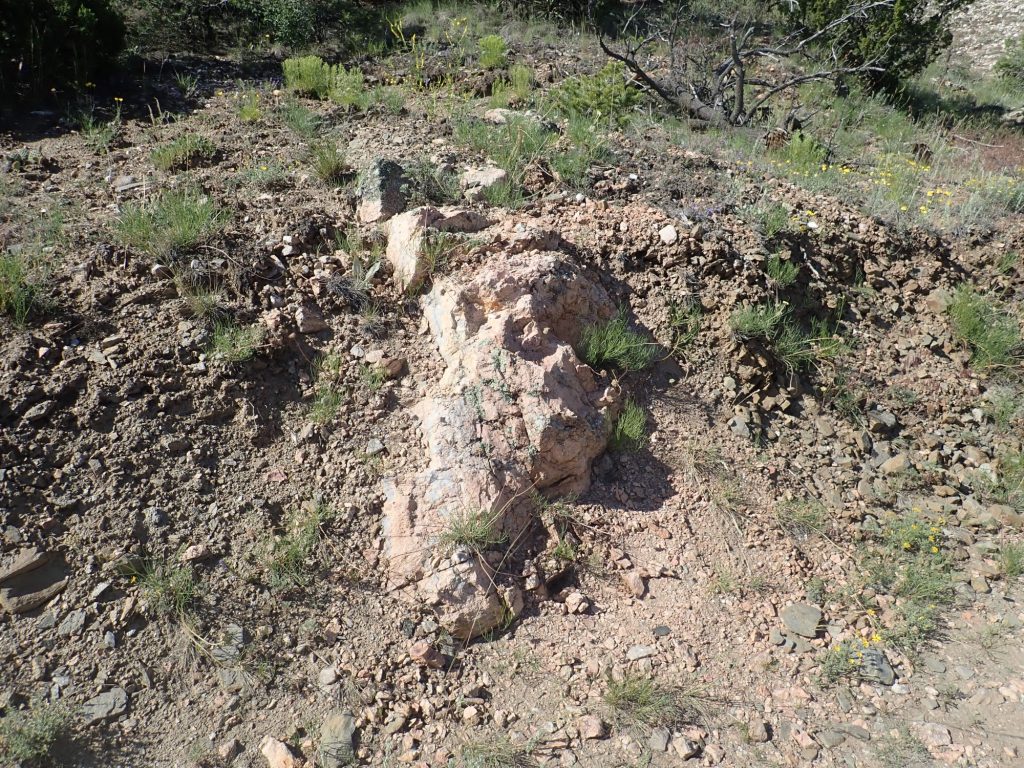
The Harding Mine pegmatite is a roughly lens-shaped body, lying on a contact beween mica schist and amphibolite, but it has a very irregular border with a fair number of small dikes coming off it. This is very likely one of them.
We arrive at the second parking area. In the distance, Cerro Pedernal.
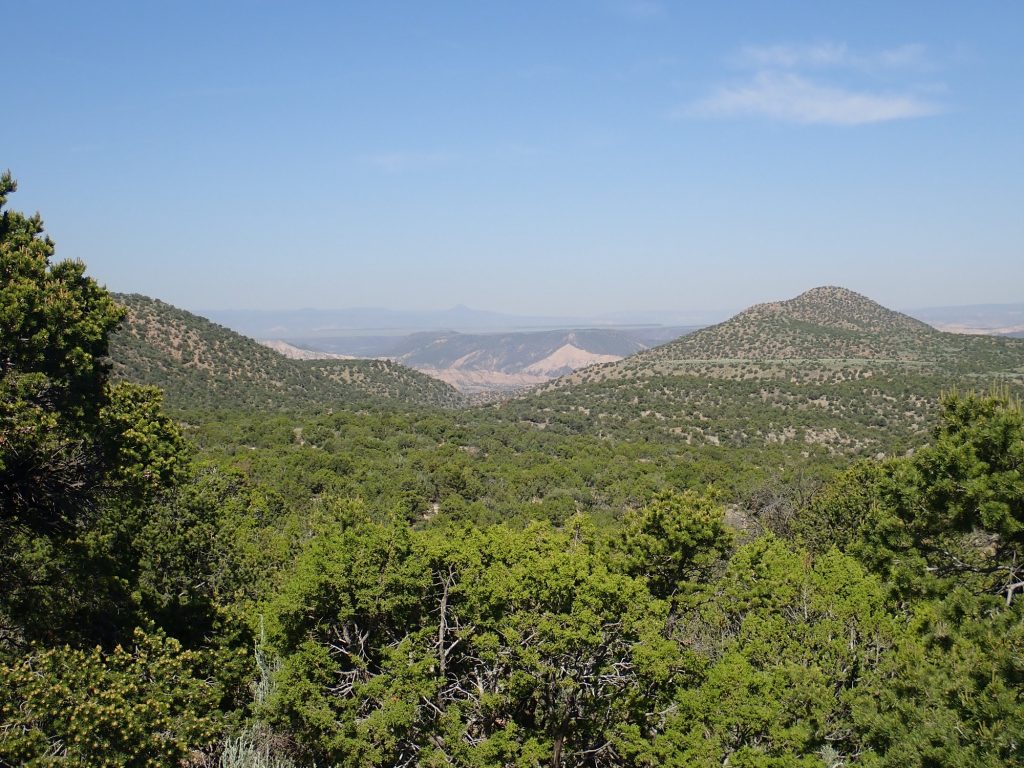
We’re looking across the Rio Grande Rift, the great crack in the Earth’s crust roughly coinciding with the Rio Grande Valley. In the foreground are hills of Embudo Granite, at least 1.3 billion years old. Beyond are tan sediments of the much younger Santa Fe Group, filling the Rift, with the black basalt cap of Black Mesa. The Santa Fe Group is about 15 million years old in this area while the basalt is about 4 million years old. Beyond are the Jemez Mountains on the west side of the Rift,with Cerro Pedernal capped with Jemez basalt around 7 million years old.
We pass through the gate. UNM (owner of the mine, which is now an outdoor geology laboratory) is good with walking visitors but is serious about keeping vehicles out. Visitors are asked to limit their take to five pounds of specimens. I got my quota last time but I do want a beryl sample.
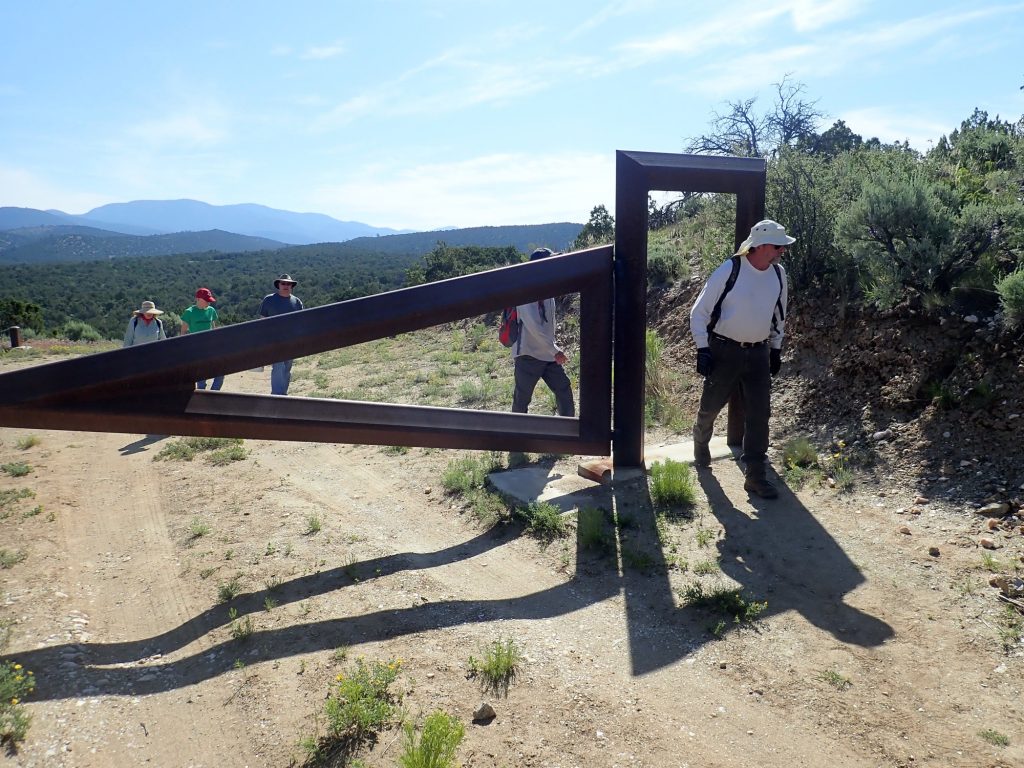
We come into view of the mine tailings.
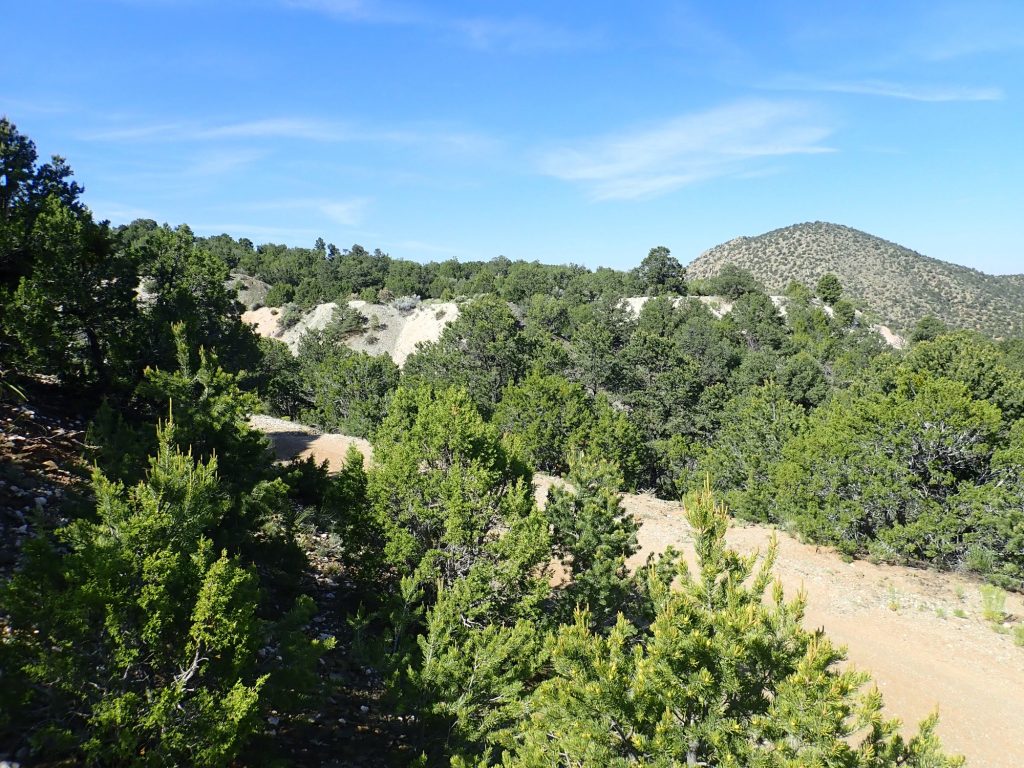
The hill at right is Cerro del los Arboles, “Hill of Trees”, which is underlain by Embudo Granite.
The outcrop here is less micaceous than the stuff earlier.
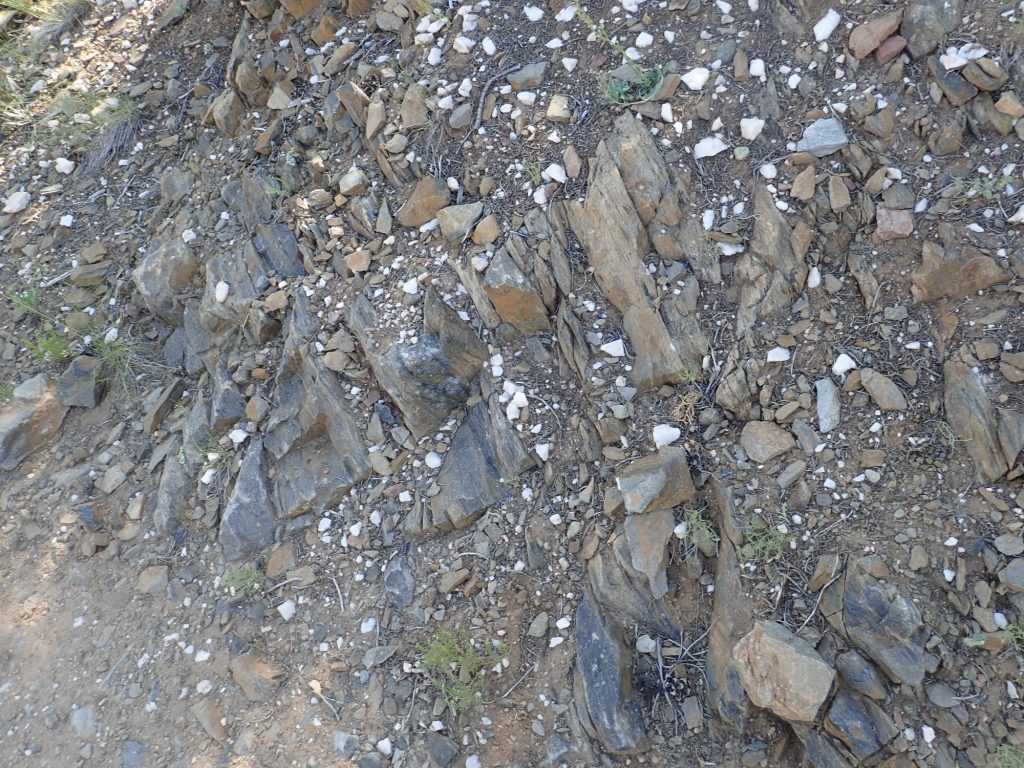
It’s still Vadito Group schist, however. Perhaps a different formation within the group.
Then we hit the main pegmatite dike itself.
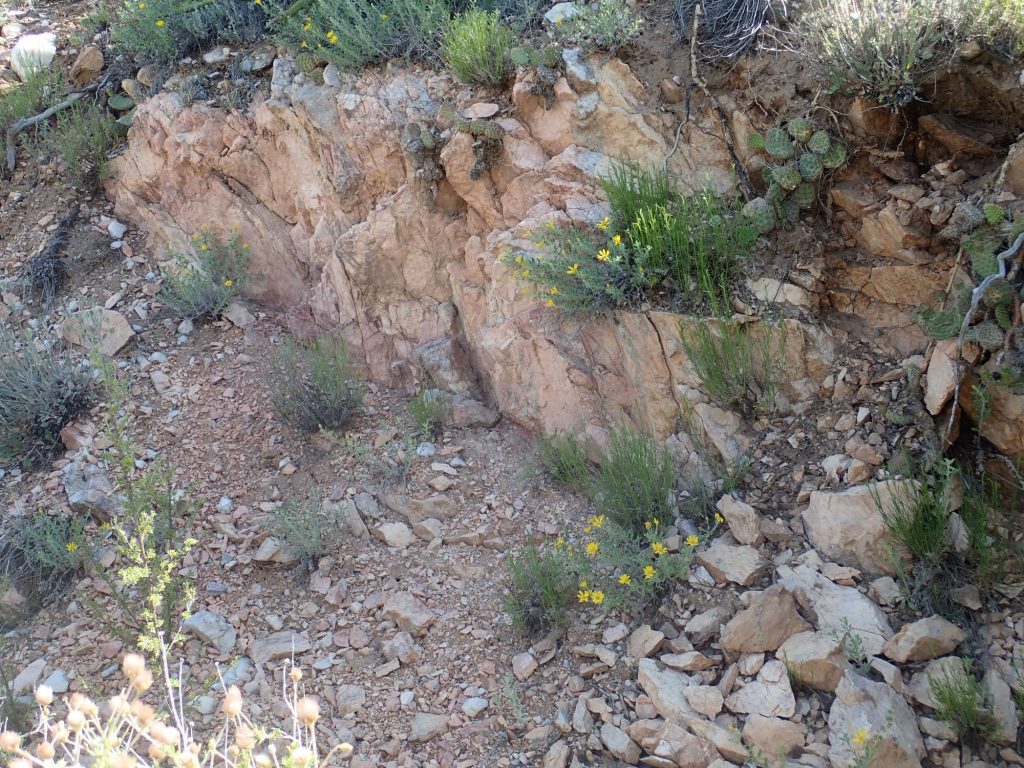
This is an outcrop of mixed albite, muscovite, and quartz making up the lower part of the dike.
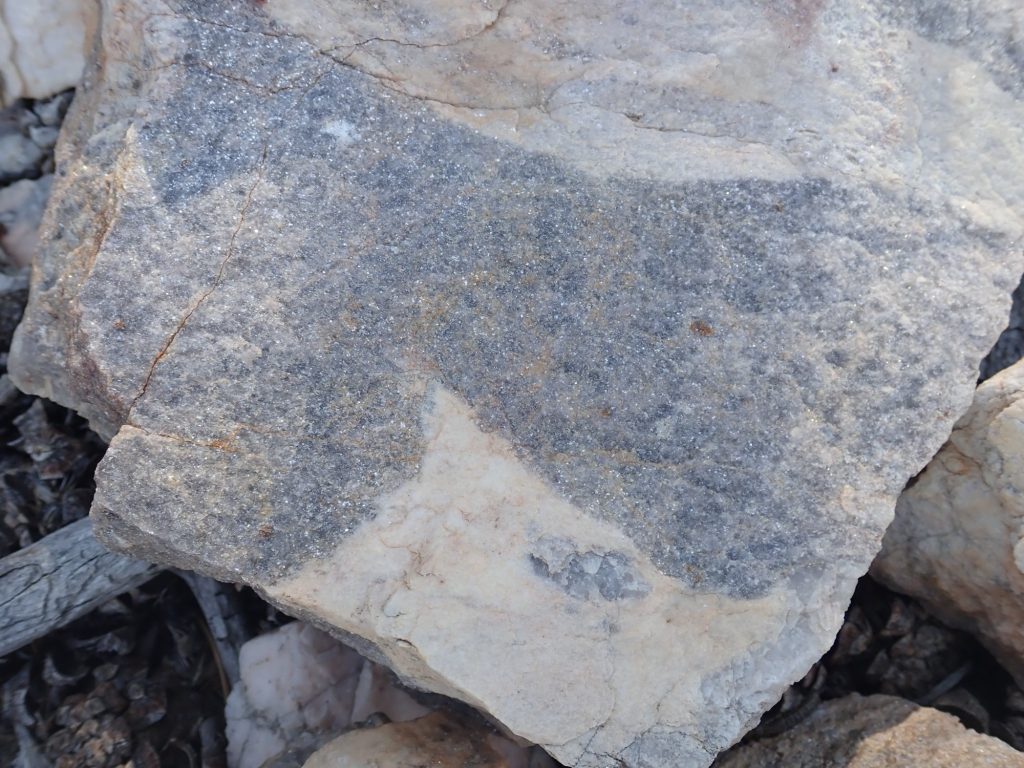
My identification is tentative; albite is not always easy to distinguish from microcline, potassiumf feldspar. But the dark gray areas of mixed muscovite and quartz are pretty unmistakeable.
Let me take a moment to talk about pegmatites and their mineralogy. If geek-talk is not your thing, just skip ahead to the next picture while I ramble.
A pegmatite is an intrusive igneous rock (one that forms from magma, molten rock, that cools while still underground) that consists of crystals larger than about an inch across. Some pegmatites are very coarse indeed, with individual crystals several feet across and weighing tons. The largest crystals I’ve seen at the Harding mine are a couple of feet long, which is plenty impressive.
Though there are rare cases of pegmatites with a low silica content, most pegmatites are composed of minerals with a high silica content, such as quartz and feldspar. In fact, most pegmatites contain little else besides quartz, feldspar, and mica, and these pegmatites are known as simple pegmatites.
The Harding Pegmatite is not a simple pegmatite. It has plenty of quartz, feldspar, and common micas, but it also has a wealth of very unusual minerals containing rare chemical elements. Mines in pegmatite outcrops are a major source of these elements, which include lithium, beryllium, fluorine, tantalum, niobium, lanthanide metals, tungsten, tin, thorium, and uranium. Not all these elements occur in the same pegmatites, and the Harding pegmatite was mined mostly for lithium, beryllium, tantalum, and niobium.
The presence of these elements is a clue to the origin of the pegmatite. They are all incompatible elements, so called because their ions have a combination of size and electrical charge that makes it difficult for them to enter the common minerals making up most of the Earth’s crust. Lithium and beryllium don’t fit snugly into ordinary minerals because their ions are tiny; tantalum and niobium are similar in size to more common elements, but their ions are so highly charged that they distort the crystal lattice too much for a good fit.
When a magma body starts to cool and solidify, the most abundant elements — silicon, oxygen, aluminum, iron, magnesium, and calcium — solidify first, because the magma is saturated with these common elements. Some rarer elements (compatible elements) easily substitute for the common elements in their crystals. For example, manganese easily substitutes for iron in most iron minerals, which is why distinctive manganese minerals are not that common even though manganese is not a rare element. The incompatible elements, which cannot easily substitute for common elements, become concentrated in the remaining liquid magma. As crystallization continues, water also becomes concentrated in the residual magma, so that the magma begins to resemble a corrosive water solution rich in incompatible elements.
This residual magma is relatively low in density, so it tends to separate from the solidifying magma and rise independently towards the surface. The Harding pegmatite has an age and isotope ratios that show it is not related to any of the large granite intrusions in the Picuris Mountains, so it likely separated from a cooling granite body that is still deeply buried. Radiometric dating shows that it formed around 1.3 billion years ago, making it one of the younger “basement rocks” of the Picuris Mountains.
The rising residual magma wedged its way upwards between schist and amphibolite of the Vadito Group that are around 1.7 billion years old. (We’ve seen some of the schist already.) It then slowly cooled and finished crystallizing. Slow cooling favors large crystals, but, in addition, the high water content of the residual magma gave it a very low viscosity. This allowed ions in the residual magma to easily migrate to join an existing crystal, rather than nucleating a new crystal. This accounts for the very large crystal sizes in the pegmatite.
Some of this is explained in the signage at the mine, which unfortunately caught a lot of glare.
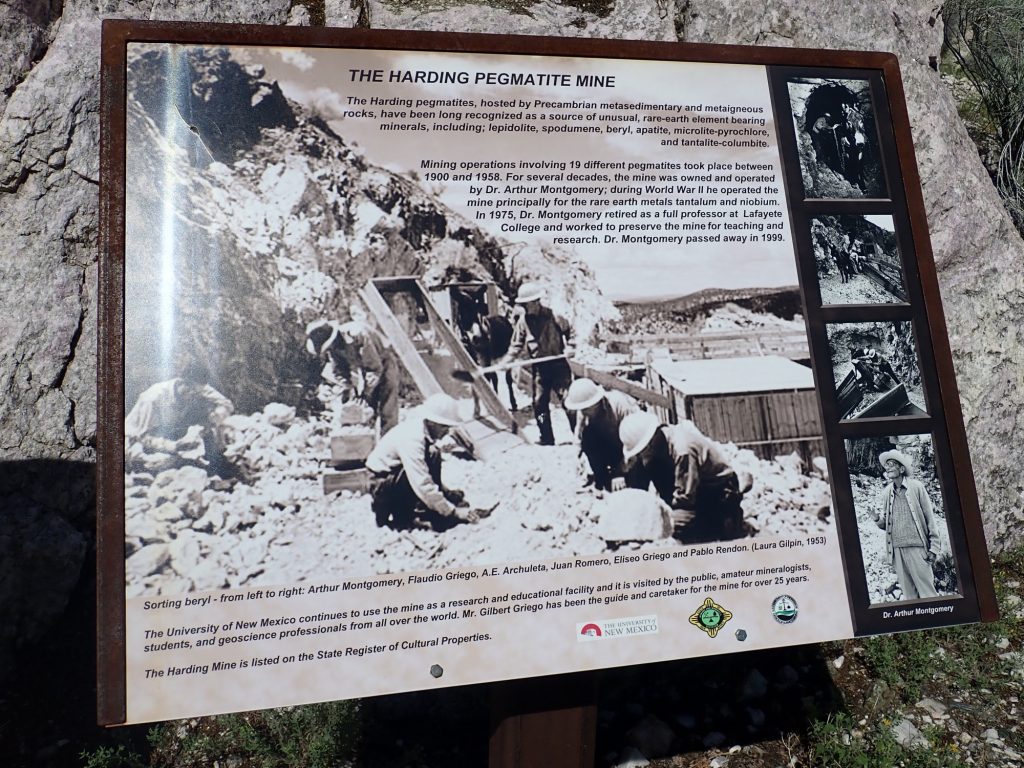
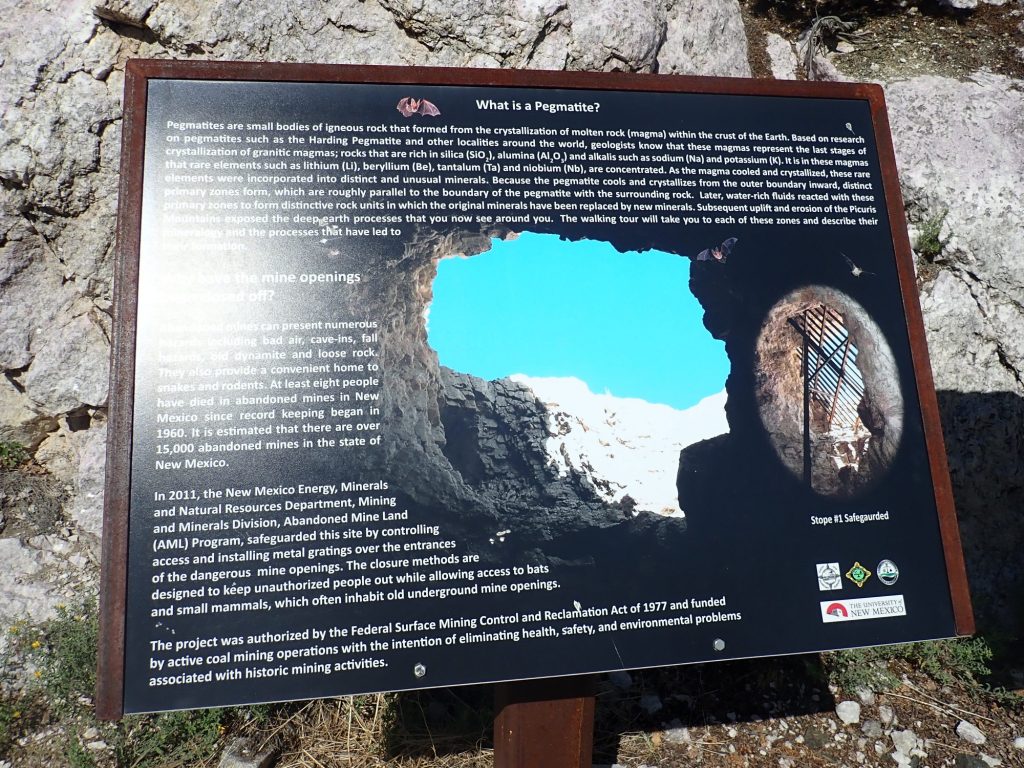
(Click to enlarge, as you may do with most images at this site.)
The pegmatite is not uniform; it is described as a zoned pegmatite. I made a point of taking the walking tour of the mine, which explains the various zones. It took me a few moments find the right trail, though other LAGS members were already ahead of me.
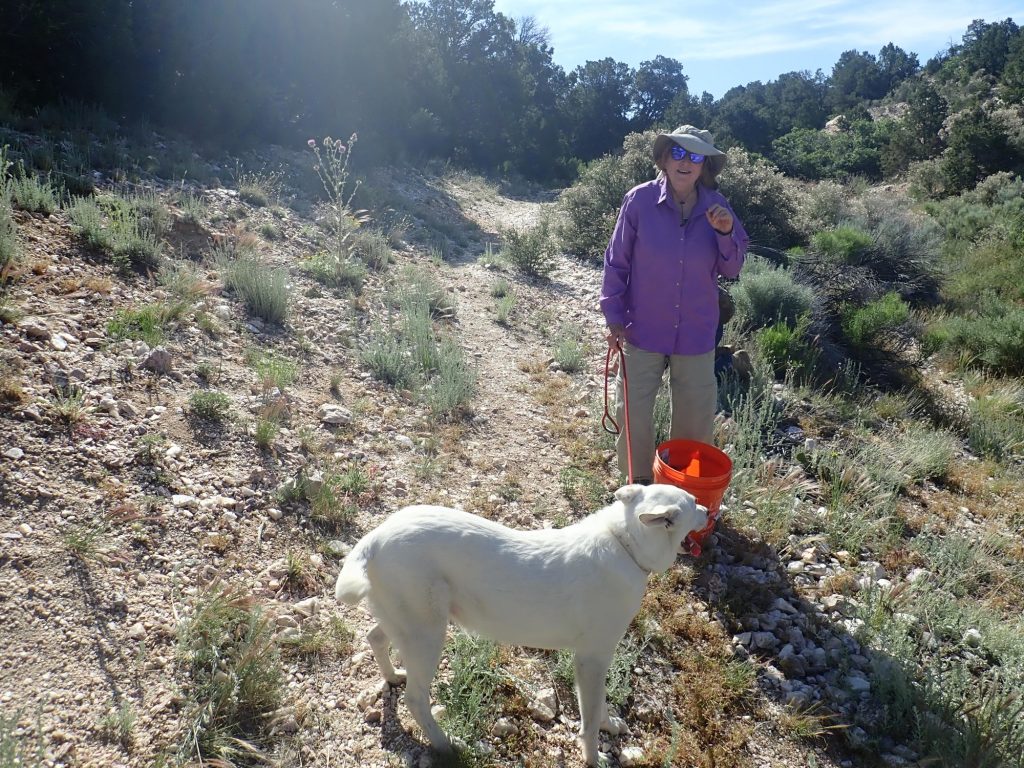
Stop 1 is on North Hill, north of the mine workings.
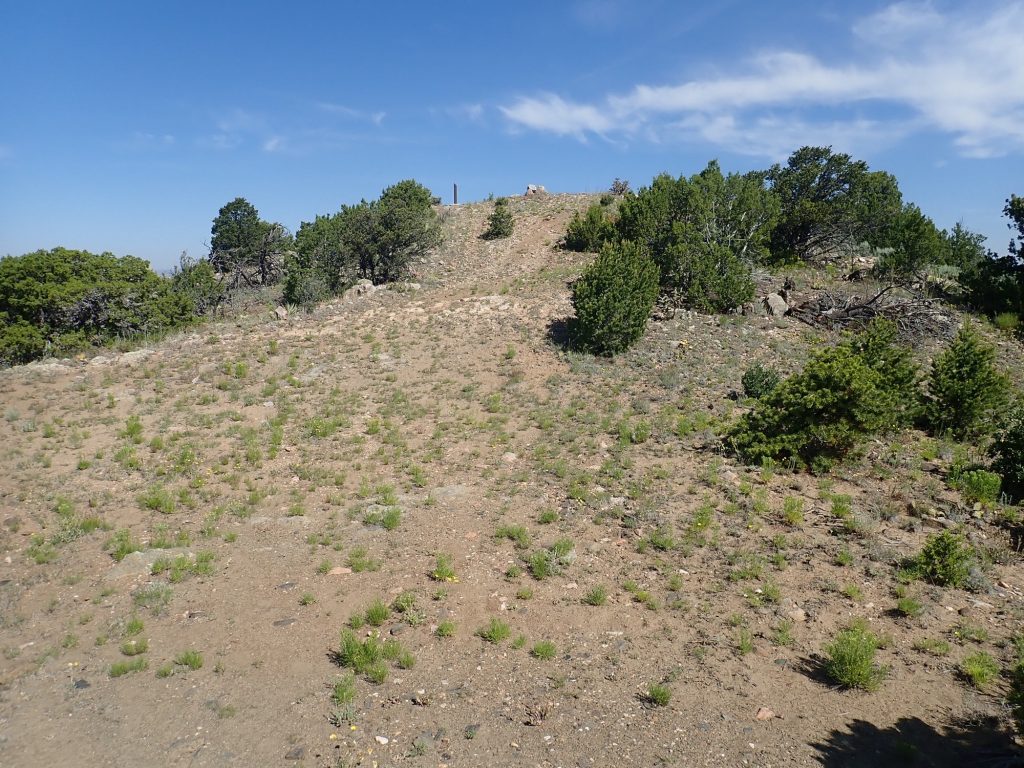
Indian paintbrush was blooming in profusion.
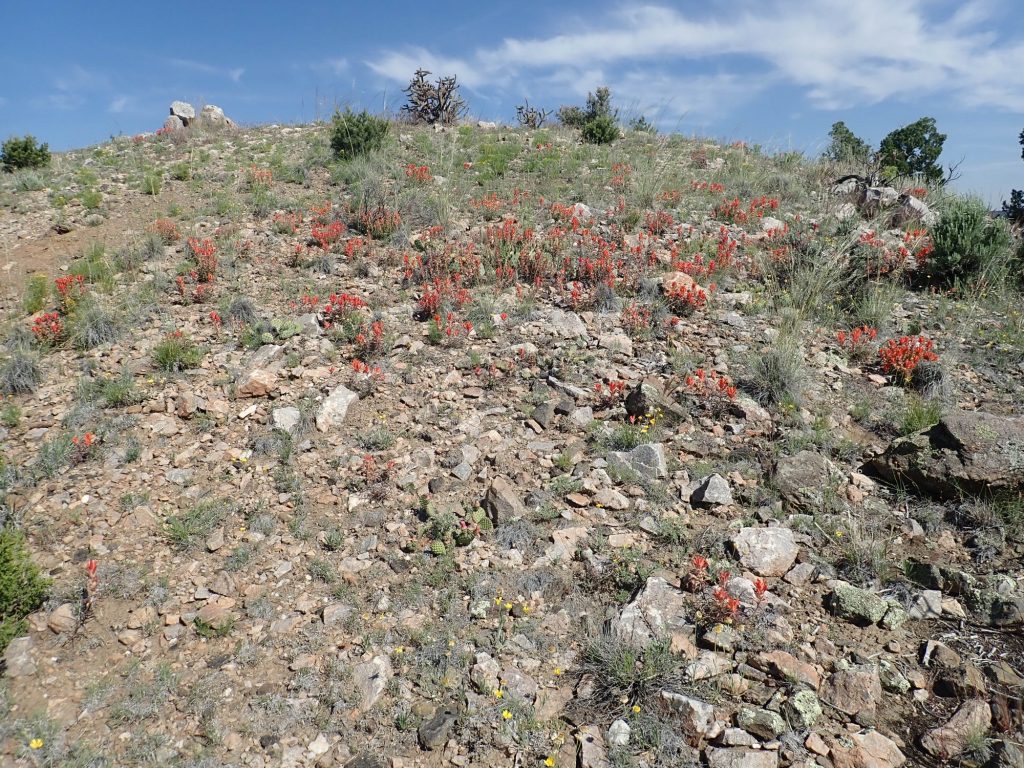
The stop gives a good panorama of the workings to the south.
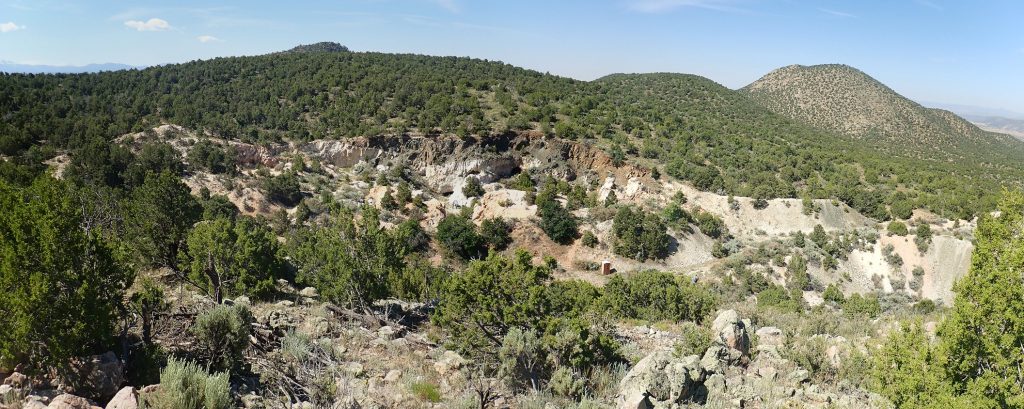
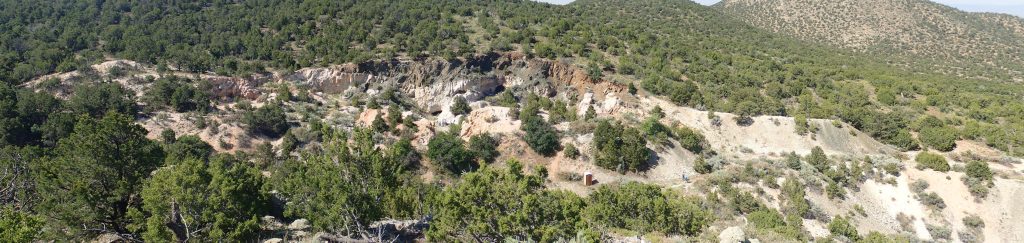
The white rock is the face of the mine. Over it is the dark amphibolite under which it intruded, forming a very sharp contact. There seems to have been very little mixing of material between the pegmatite and the surrounding rock.
The pegmatite body itself is highly flattened, and dips slightly to the south almost in the direction we are looking. The south face of North Hill is covered with remnants of pegmatite, and drilling shows that the dike behind the face of the mine extends back into the hills for 500 meters. To the east (left) the dike becomes a very irregular mass of swells and branches.
Rock cairn (of schist boulders) at the hill top.
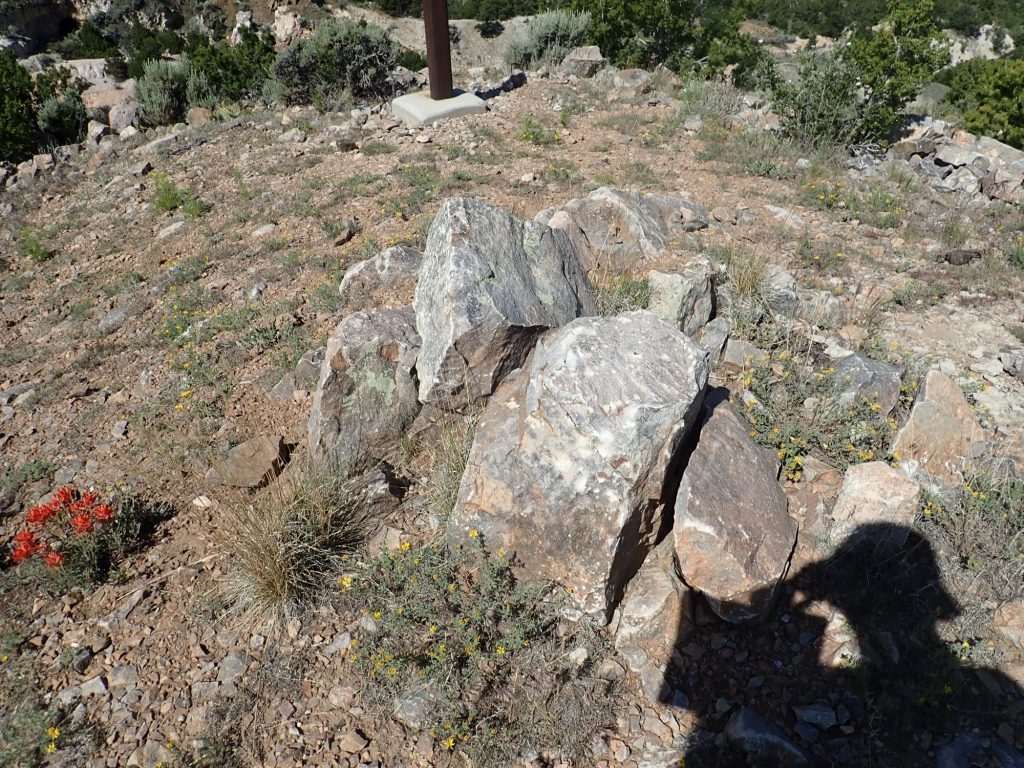
I headed east to the second stop, pausing for some very odd-looking rocks.
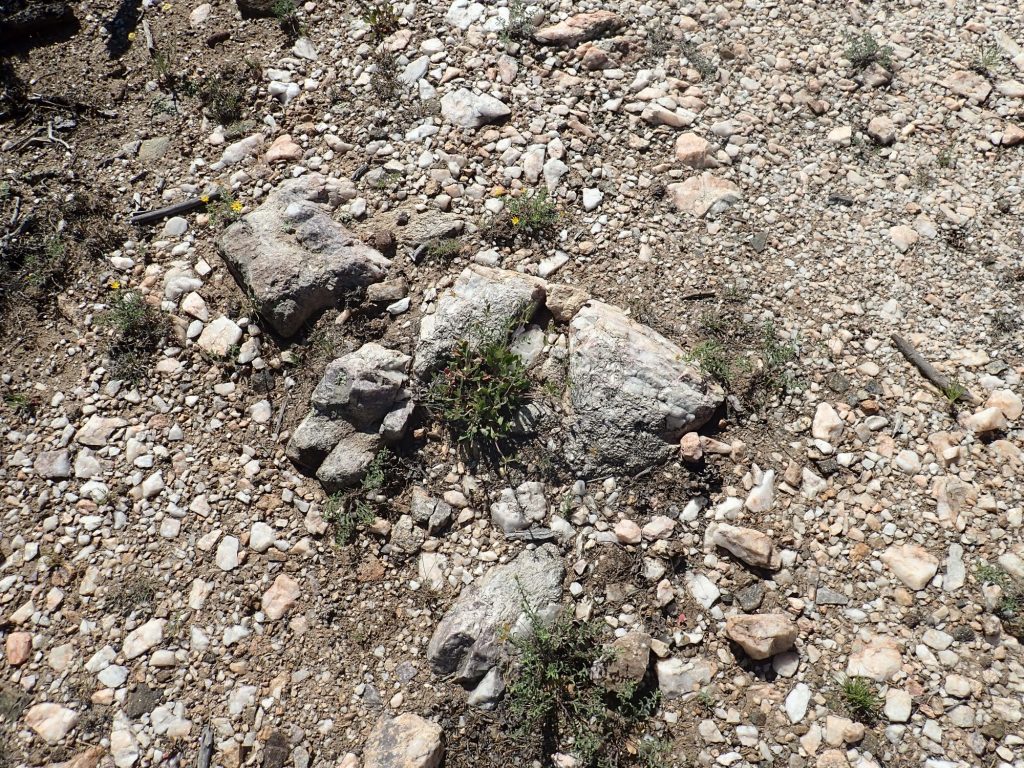
I’m not bad at reading a map, and I arrive at an area that fits the description in the walking tour guide perfectly. But I can’t find the signpost.
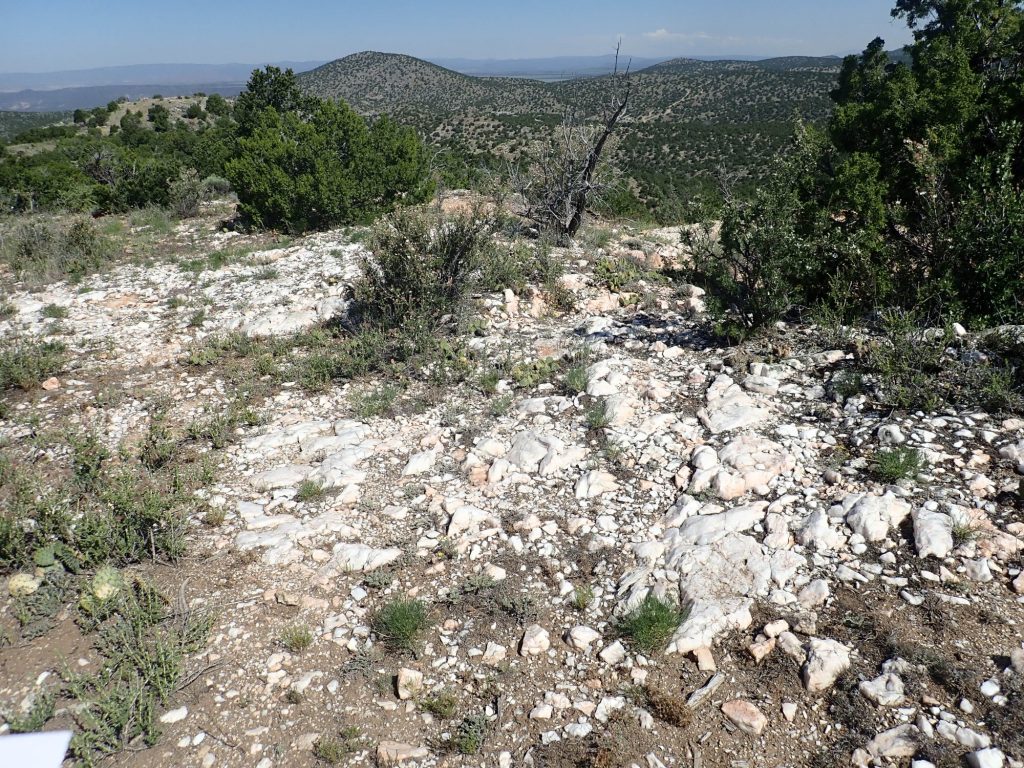
This is the upper surface of the dike, exposed by erosion of the overlying amphibolite. And here is the prospect pit mentioned in the guide.
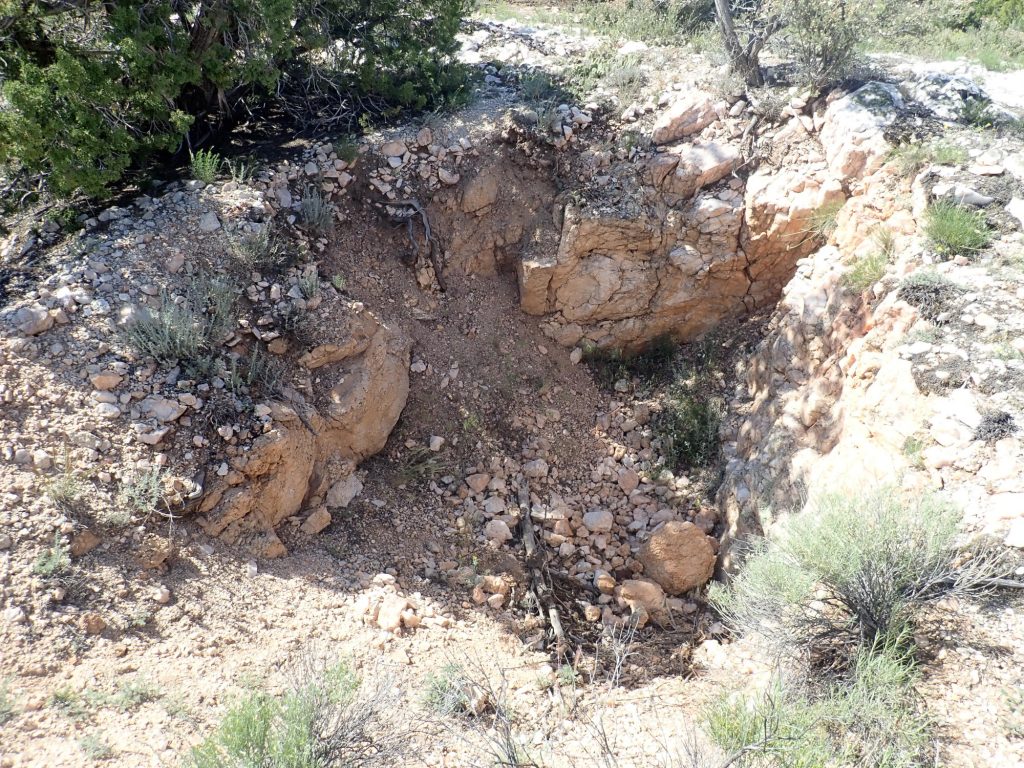
It’s partially eroded away here, but the uppermost zone of the mine is a thin rind of relatively fine-grained quartz, albite (sodium feldspar), and muscovite mica, then a thicker, coarser layer of the same minerals plus (in some locations) accessory minerals such as microcline, apatite, lepidolite, tantalite-columbite and beryl. The beryl mined at the west end of the workings came from this berry zone. Below the berry zone is a layer of more or less pure quartz.
Also visible near this location is clevelandite.
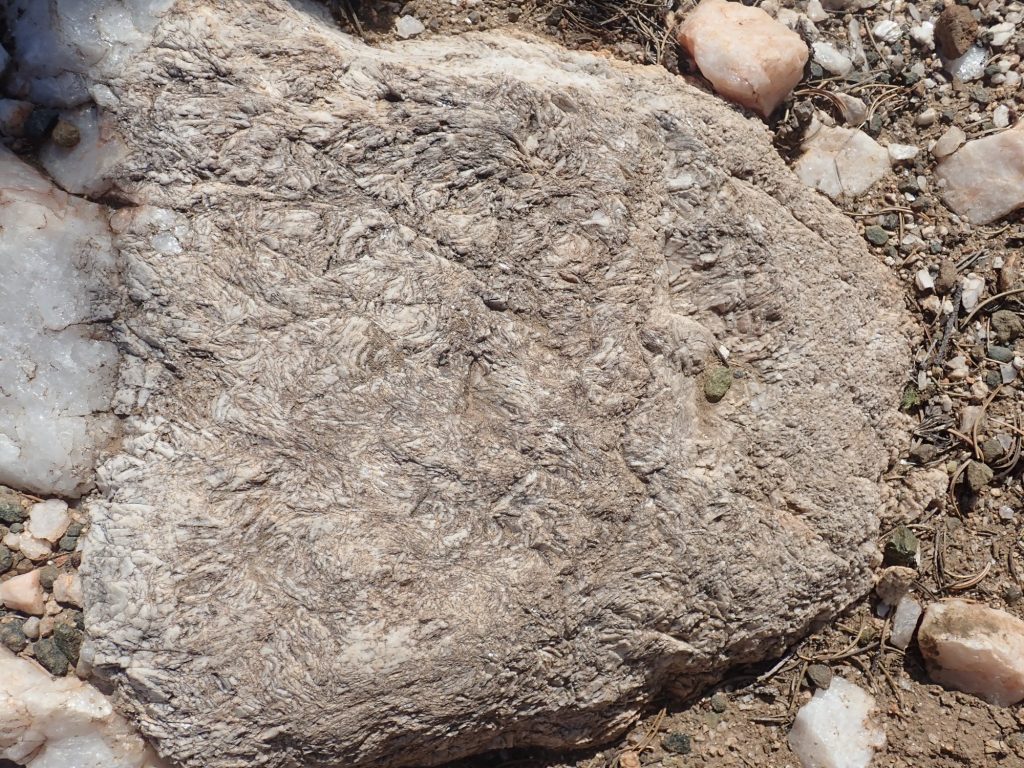
This is a form of albite with peculiar curved platy crystals. It tends to cut across other zones in the pegmatite, but is more typical of the center of the dike. It’s what I first spotted about three photos back. It’s unusual enough that I took an unplanned sample.
I wander around a bit, then head back to this area, and finally spot the #2 signpost — at the east end of the quartz outcrop, almost invisible against the trees.
It’s a bit of a scramble to the third stop, which is high above the east end of the mine.
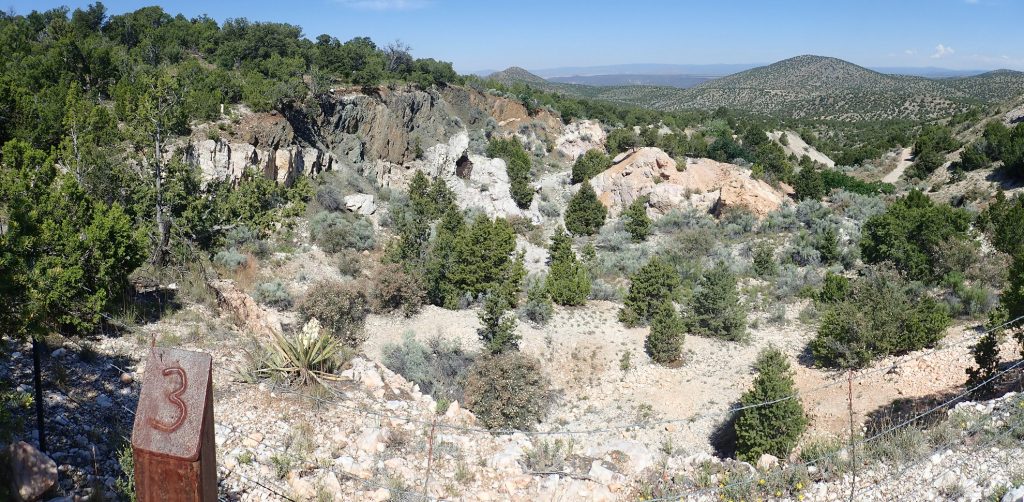
We’re looking into the main mine workings. Mining was apparently mostly by primitive means and pretty haphazard. Here we’re standing on the quartz zone; the berry zone has been eroded away.
At center right is a big knoll of “spotted rock”, which is more or less the central zone of the dike.
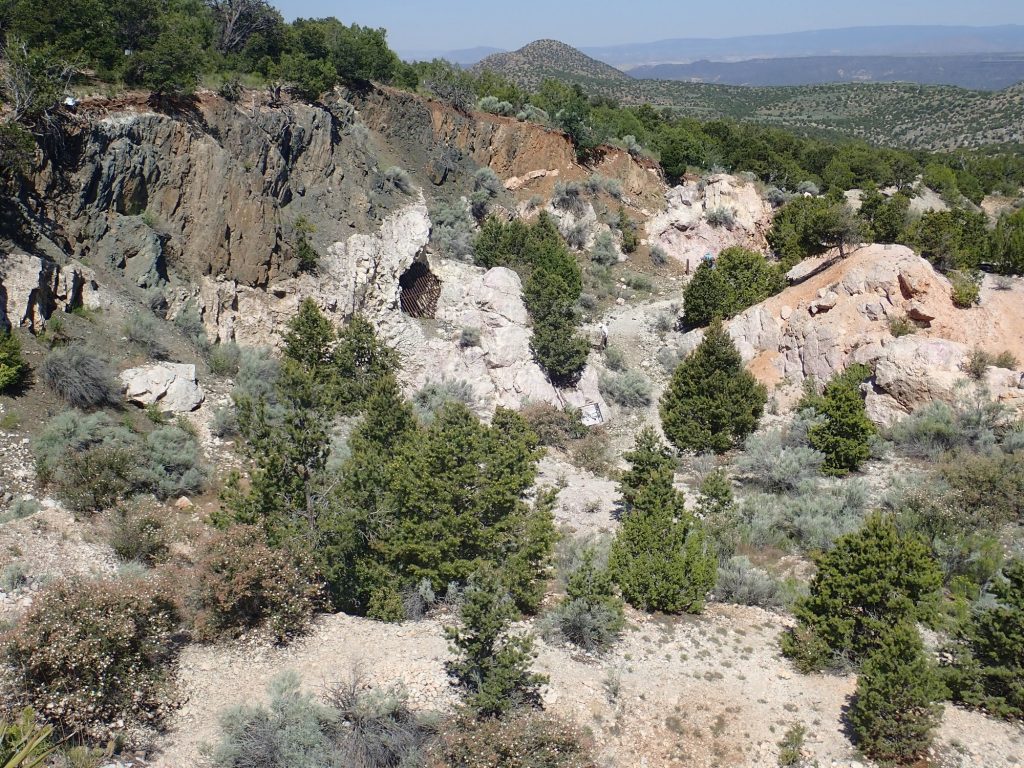
We’ll get a good look later.
I work my down into the mine workings to Stop 4, at the east end of the mine. On the way down, I pass some shallow workings at the base of the quartz zone.
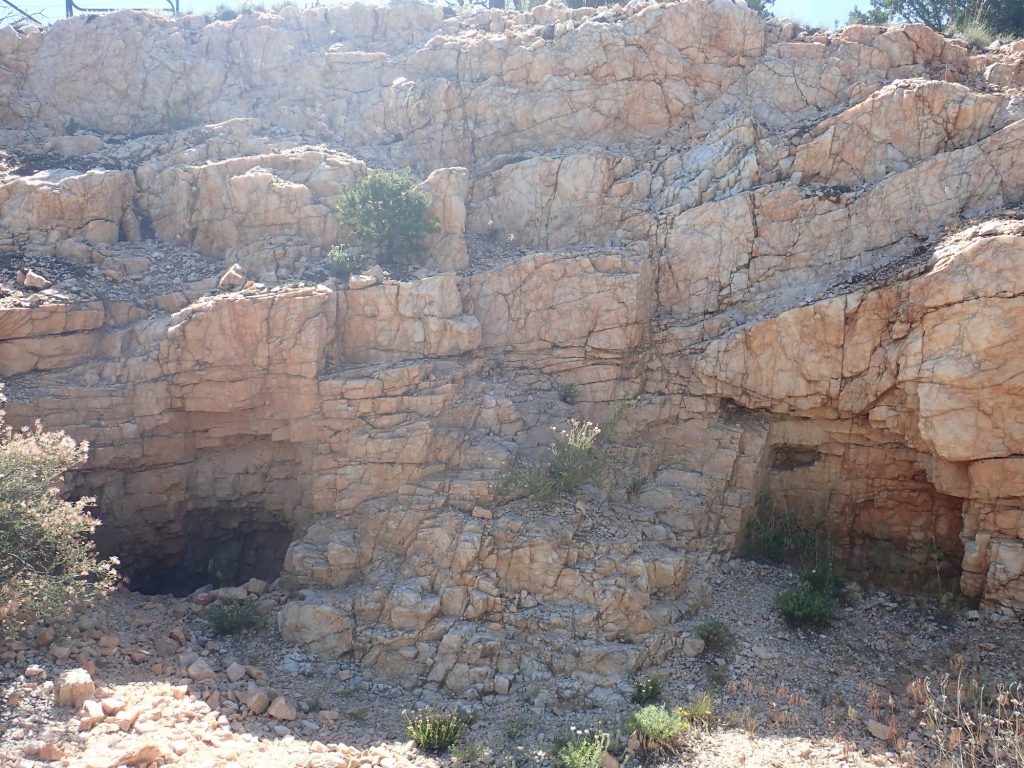
These likely were looking for spodume, a lithium aluminum silicate of the pyroxene family. Spodume occurs as amazingly large white crystals — a little like sections of 2 by 4s in size and shape — in the next zone, the quartz-spodumene lath zone. Spodume was one of two principal lithium ores in the mine,the other being lepidolite. My impression is that this area once had these crystals but has now been picked completely clean.
Below this area is the rose muscovite-cleavelandite zone.
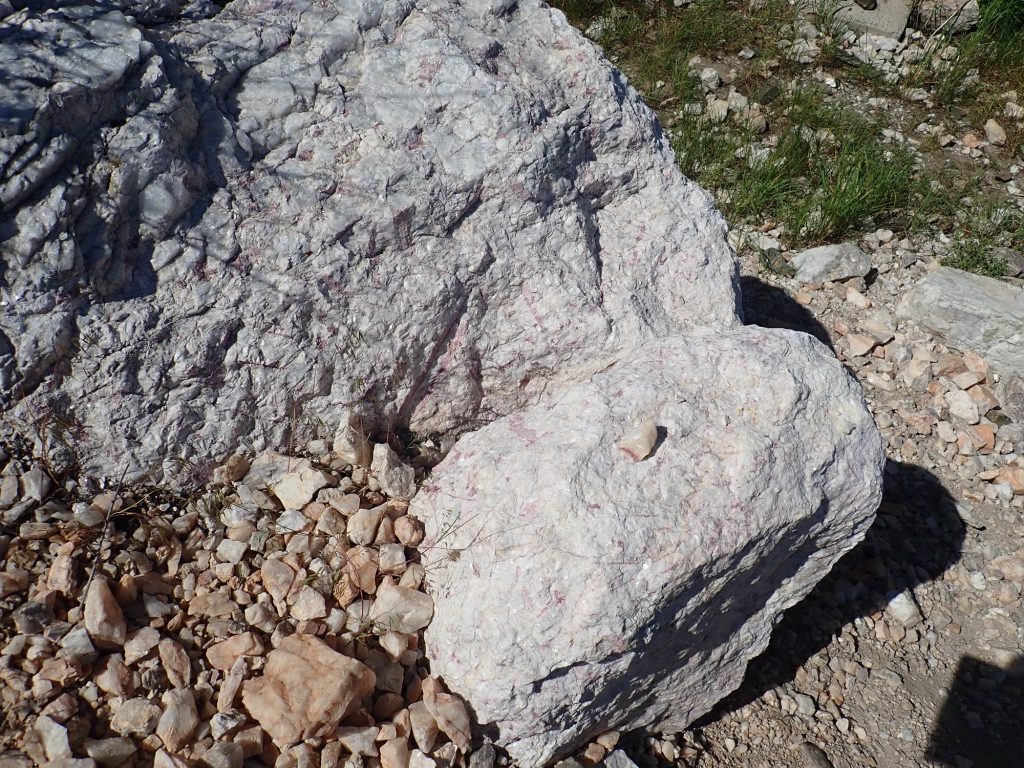
This zone is not a solid sheet, but forms pockets throughout the central dike. It is a replacement zone. This is seen better at Stop 4:
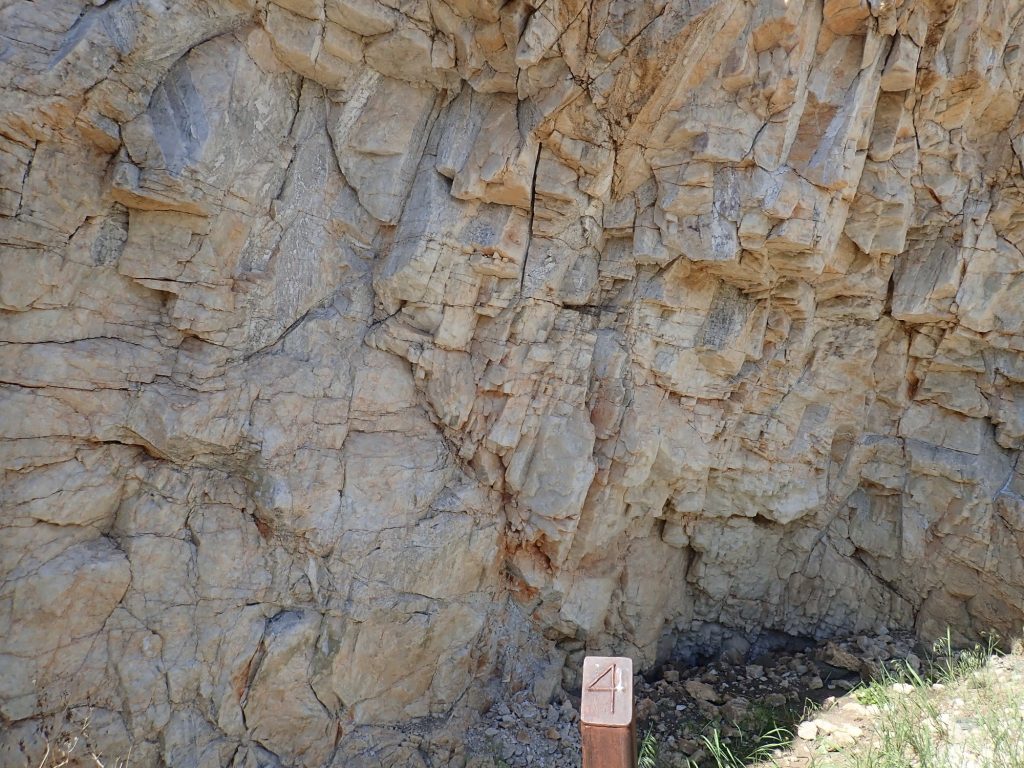
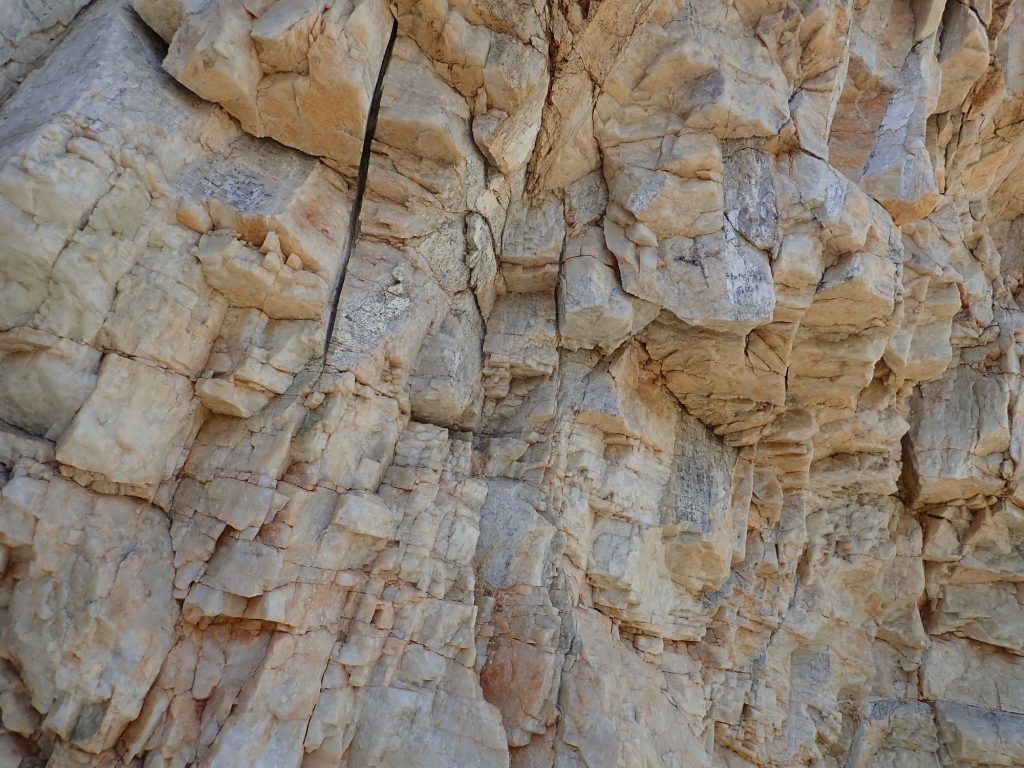
As the residual magma became more concentrated, it began reacting with the zones deposited earlier. Here, quartz was replaced with cleavelandite as sodium aluminate in the magma reacted with it. Spodume was replaced with rose muscovite as potassium reacted with. The rose muscovite still has substantial lithium content.
Replacement was not complete, and it was once still possible to find impressive spodume specimens here. They’ve been well picked over at this point.
The “spotted rock” knoll in the center of the workings.
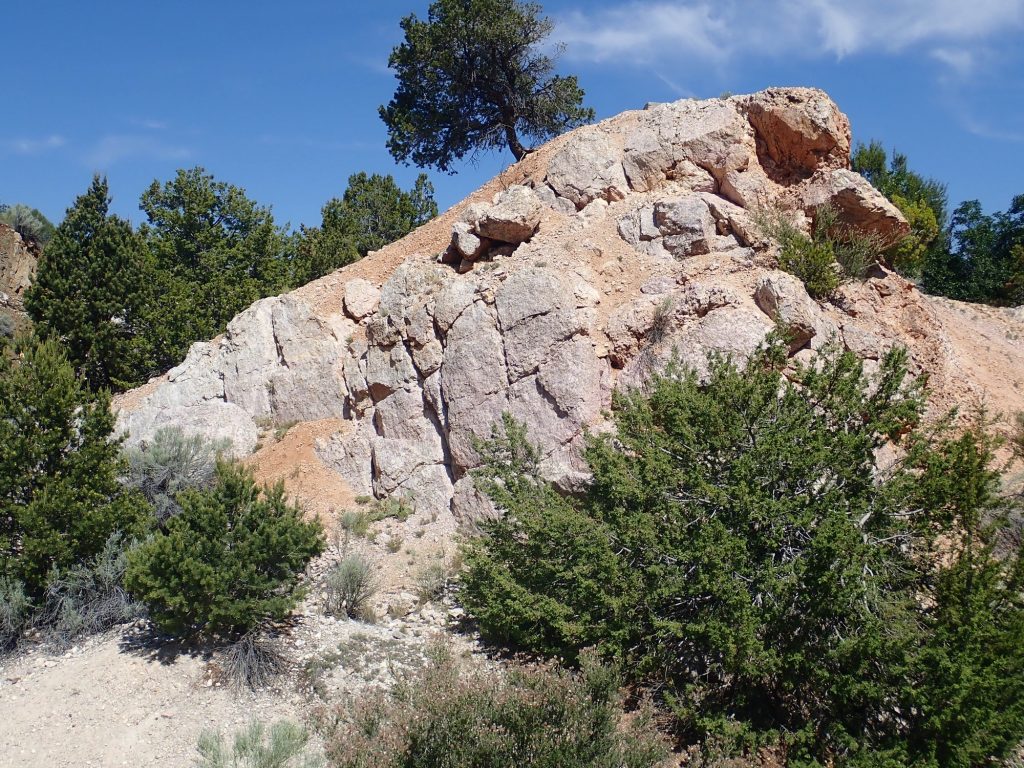
Stop 5 is near here.
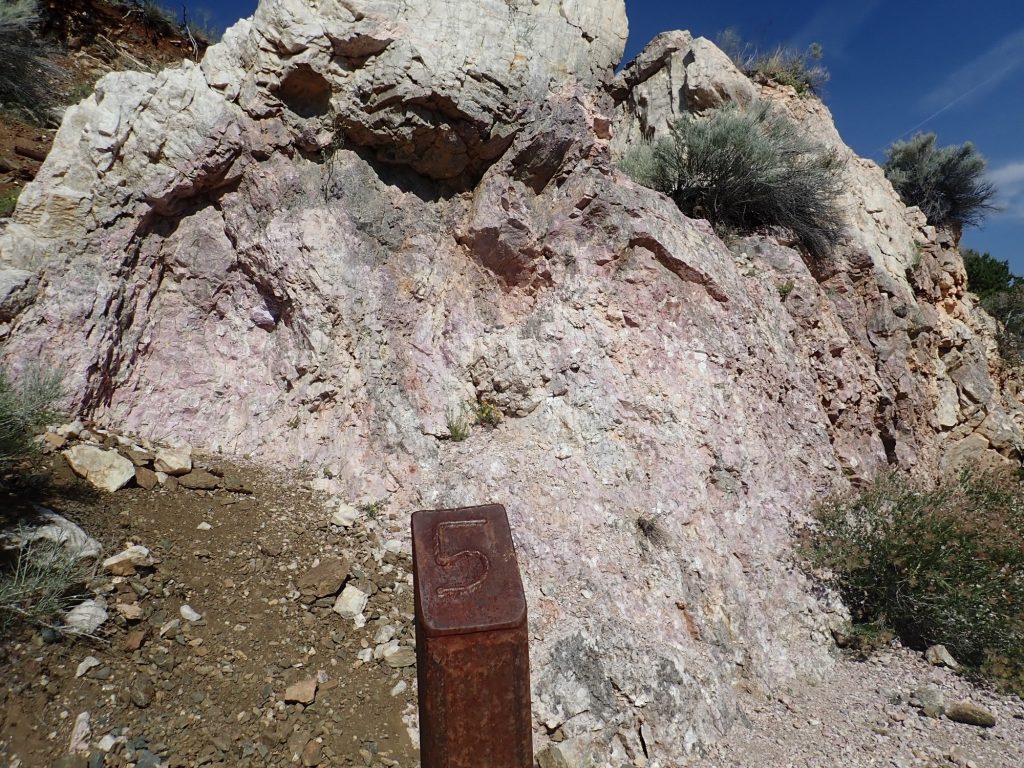
And the pink face is a fine outcrop of “spotted rock”.
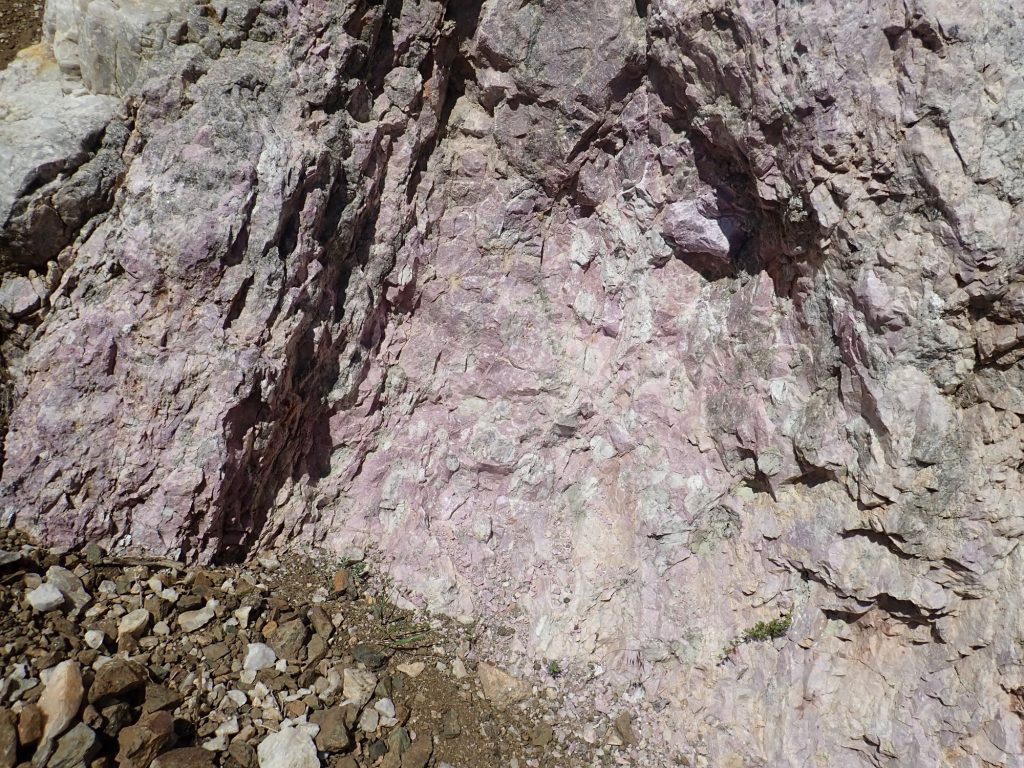
According to the walking tour pamphlet, the white “eyes” are spodumene, and the pink is microcline, potassium feldspar, impregnated with lepidolite and lithium muscovite (two forms of lithium mica) that give it a pink coloration. Close view:
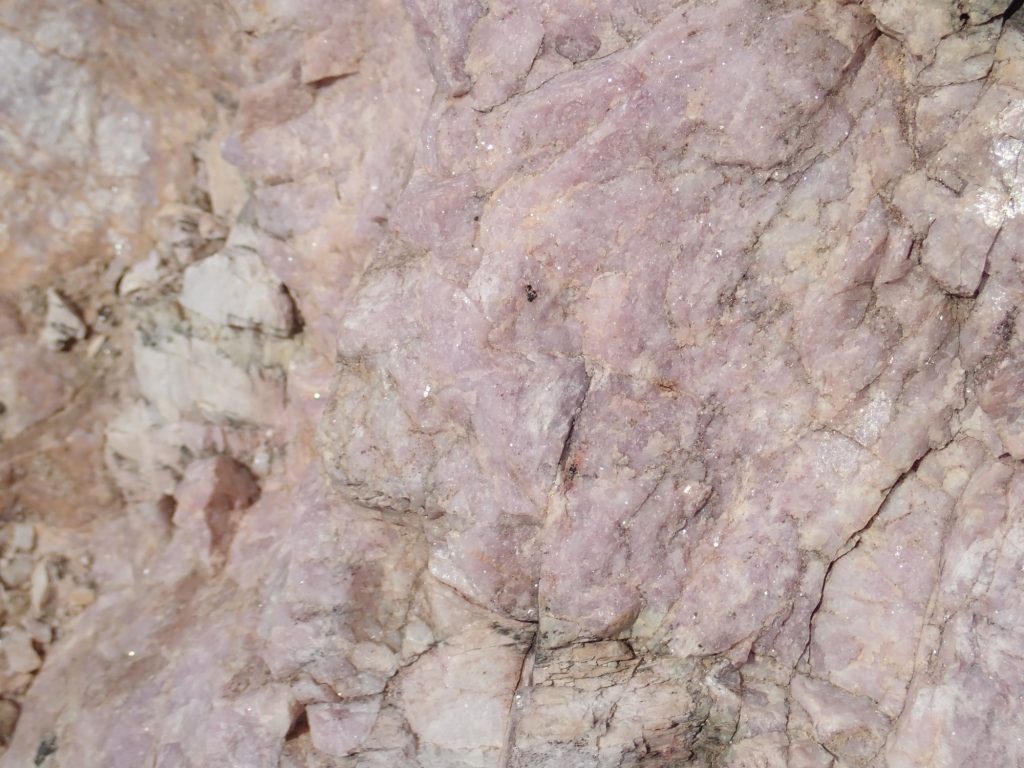
The shaggy patch at bottom does indeed look like poorly crystallized spodumene. So does the patch left of center. The tiny patch just above center is probably microlite (not to be confused with microcline), one of the tantalum minerals in the mine, which also contains significant uranium. Had I known, I could have taken it as a sample, but I didn’t notice at the time. Microlite forms larger crystalline masses higher up, at the base of the quartz-spodumene zone, but most of the good stuff was mined out and the rest picked over by collectors.
The lepidolite-impregnated microcline actually forms sizable crystals, as judged from the cleavage faces. The crystals actually grew around the much tinier crystals of lepidolite and lithium muscovite.
The crummy, rounded crystals of spodume are likely resorbed. The spotted rock formed where the increasingly concentrated residual magma reacted with zones that had already solidified. Here the magma ate away the entire quartz-spodume zone, but the spodume crystals were only partially dissolved, leaving smaller and rounded spodume patches.
I step back and get a look at the rock pinnacle here.
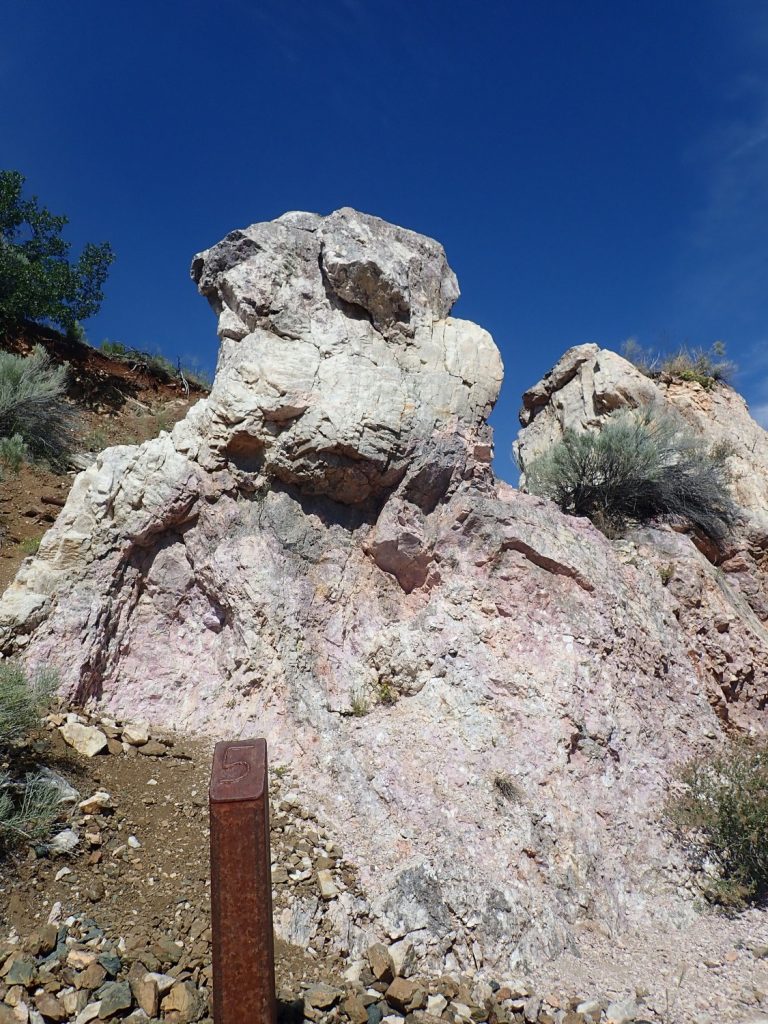
This is probably the best spot to see the zoning. The top of the pinnacle was the upper surface of the dike, from which the amphibolite has been eroded. The gray cap is the berry zone. The lighter zone below and curving around to the left is the quartz zone. There is little left of the quartz-spodumene zone except a region of more abundant mica in the upper part of the spotted rock.
I am only rapidly skimming my walking trail pamplet, but it says there is beryl in the wall zone here. I haven’t yet understood that the wall zone is a synonym for the berry and quartz zones, and I am left uncertain where I’m supposed to find the beryl. I scout the area and chip a few samples, but when I show them to Patrick Rowe later, he will confirm my fear that my samples are just feldspar. Beryl is very difficult to distinguish from quartz or feldspar.
I climb up to look at the top of the pinnacle. There are bluish-green crystals of apatite here.
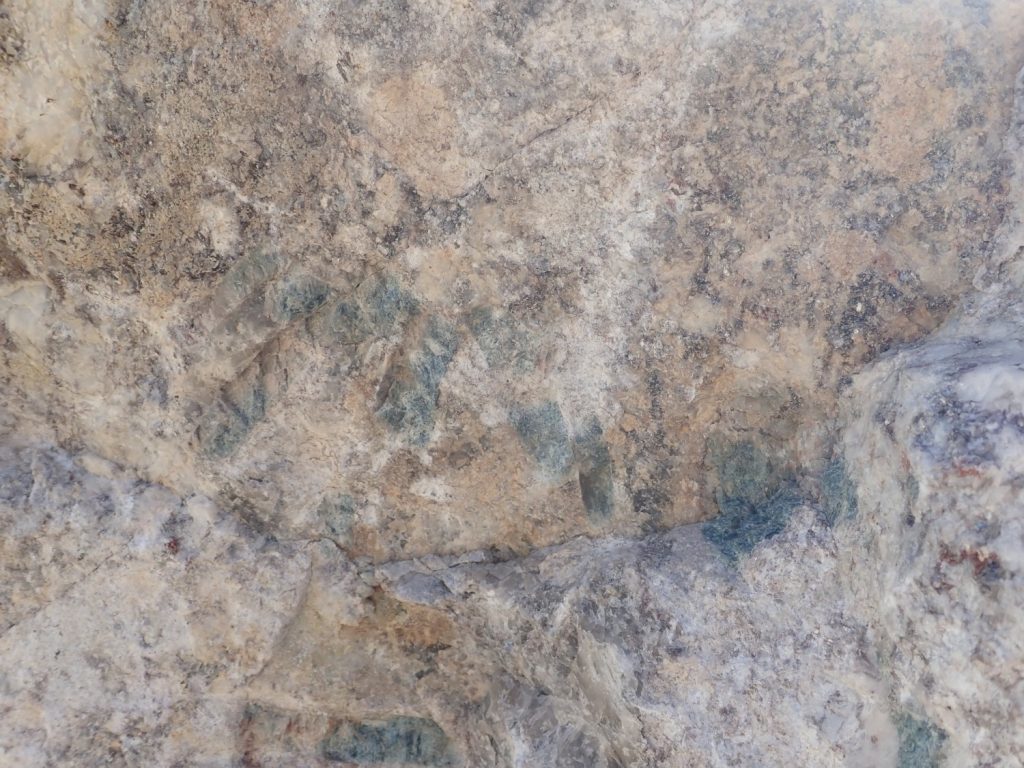
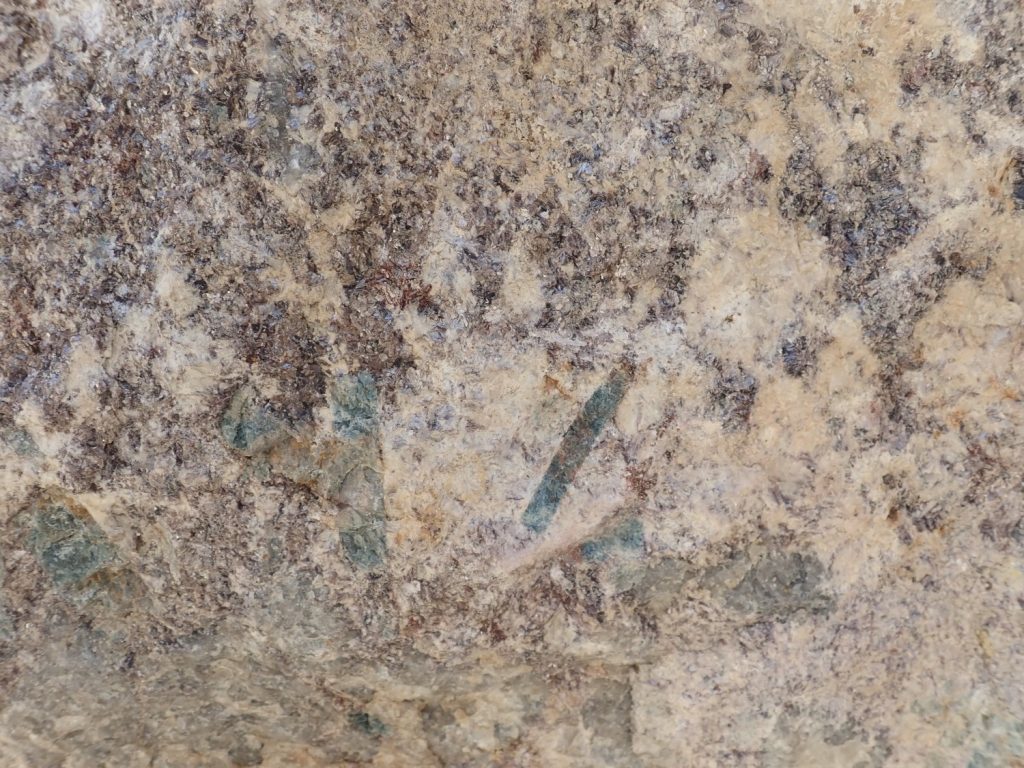
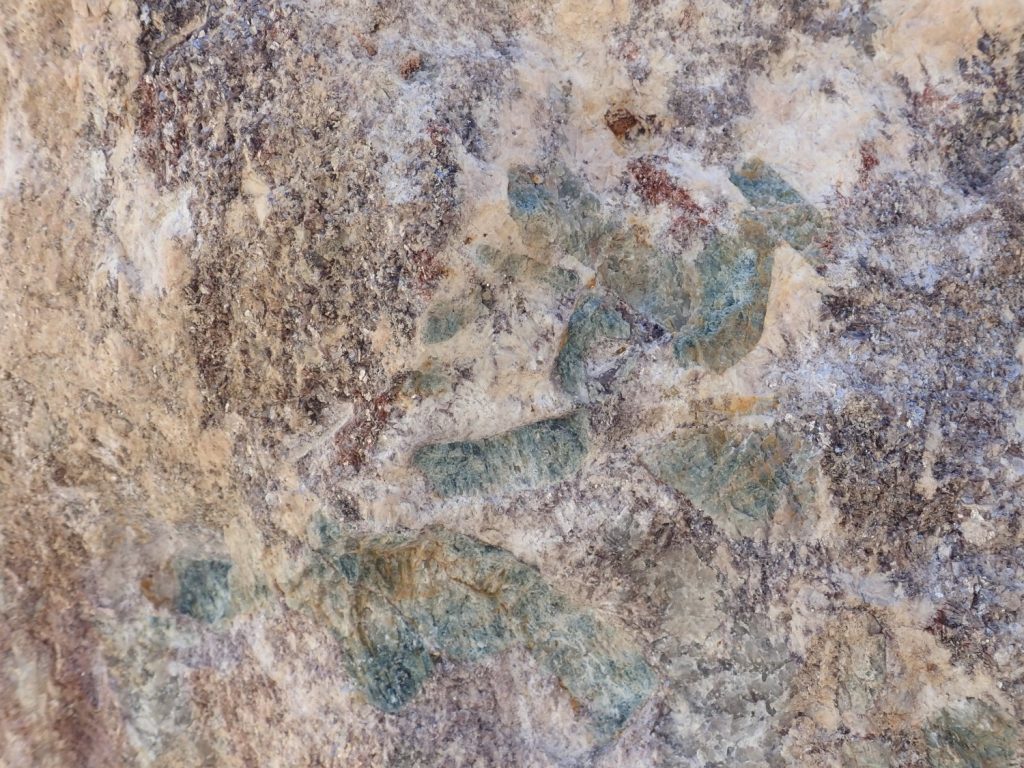
Apatite is a hard substance (the enamel of your teeth is chemically very similar) but not as hard as the silicate minerals here (except mica.) This means there is little hope of hammering loose an intact crystal. However, I already have some good apatite samples from earlier visits. They fluoresce a brilliant mustard yellow under shortwave UV light.
I don’t realize it, but this is the berry zone and I’m probably looking right at some beryl here. I fail to distinguish it from the feldspar and quartz.
Sixth stop on the walking tour, just a few feet northwest of the fifth stop.
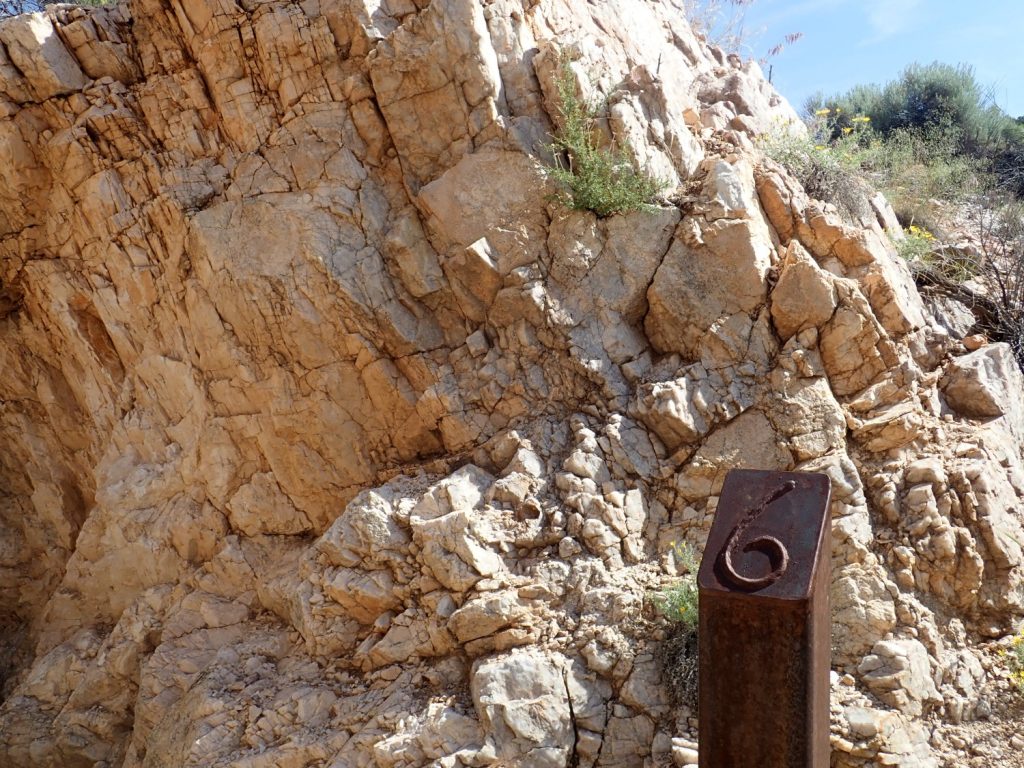
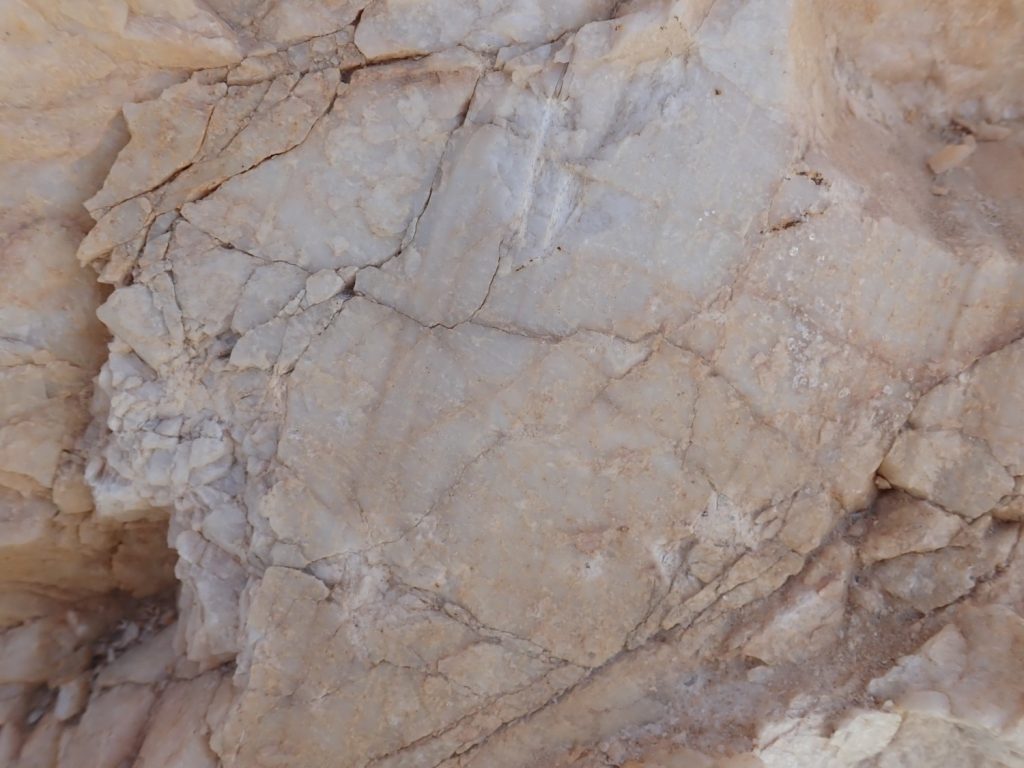
Perthitic feldspar. This is mixed sodium-potassium feldspar which, as it cooled, separated into thin alternating layers of albite (sodium feldpar) and microcline (potassium feldspar) with the latter likely predominating. As zones of minerals solidified on the top of the dike, other mineral zones formed on the bottom, and this is the second zone that formed on the bottom. Much of it was altered to spotted rock, but his outcrop was left intact.
I find Patrick Rowe and ask him to show me some beryl so I’ll be able to identify samples. He hands me a cobble with a large patch. For the life of me, I can’t tell it apart from quartz. It’s supposed to have a distinctive greasy luster, but I just don’t see it.
At Patrick’s suggestion, I go to the tailings pile at the west end of the mine, where beryl was most extensively mined.
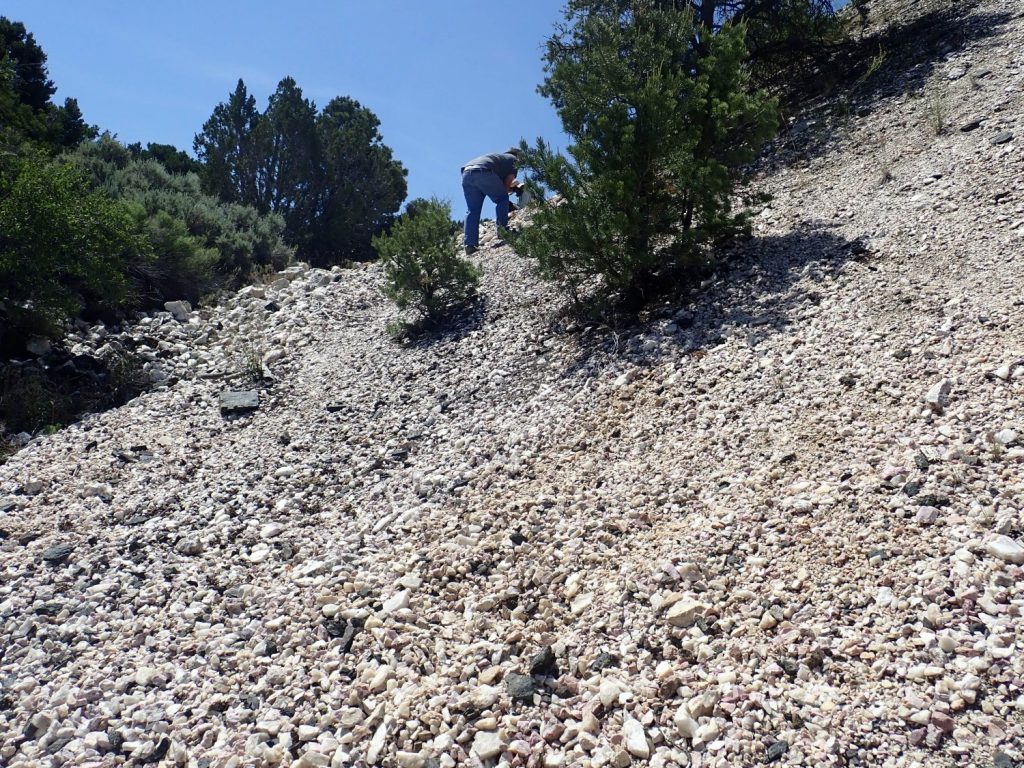
I find several samples but fear they are all quartz; alas, when I hunt down Patrick again, he confirms my fear. Frustrated, I take the cobble he showed me earlier, hammer it apart, and get some samples from the beryl patch. Now I see the difference: It looks a lot like quartz, all right, but it has subtle cleavage, like feldspar. Quartz has no cleavage. It’s distinguishable from feldspar because it is more translucent and does not have the porcelain-like luster of feldspar. I think I could possibly identify beryl now, maybe.
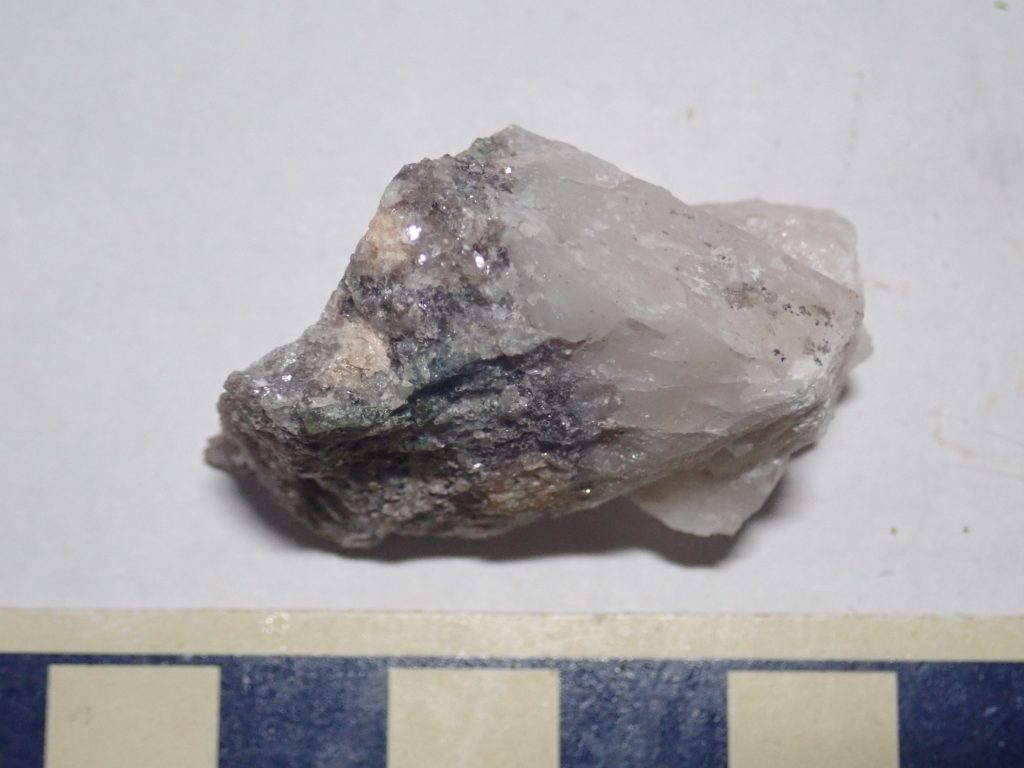
Beryl at right. Muscovite (flaky), apatite (blue-green), and spessartine (magnesium garnet; pink) at left.
One other thing I want to do is look for placer tantalite in the arroyos below the mine. I scout around a while but no luck. Well, except for some wildflowers.
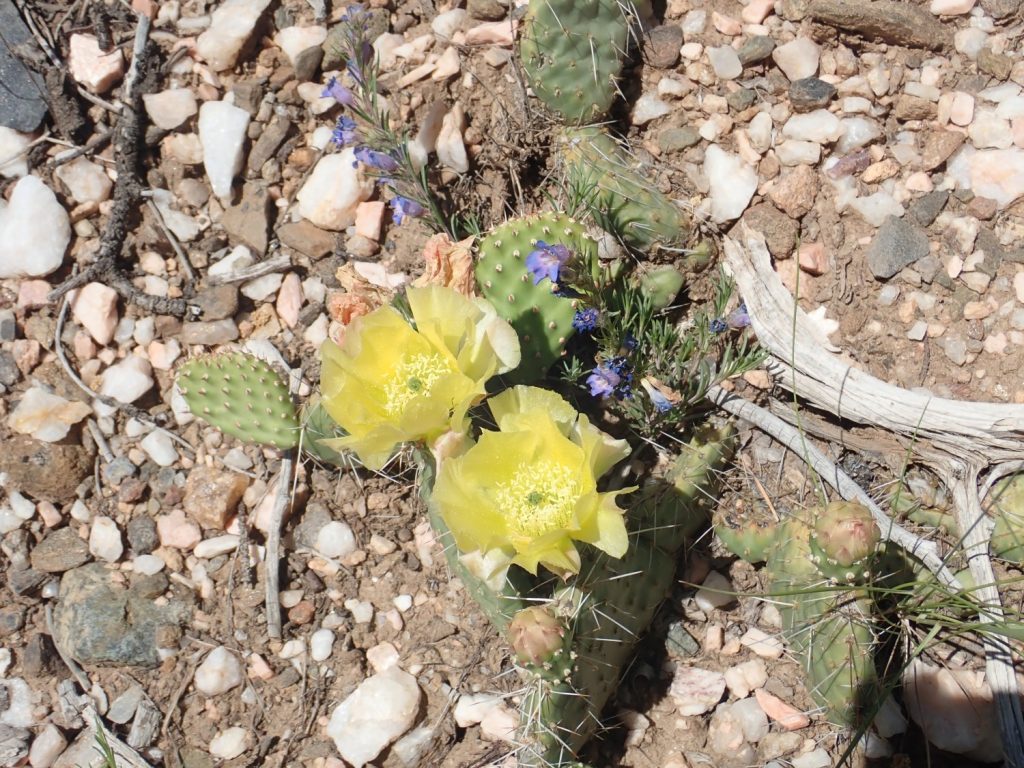
On the way back to the mine, I find myself in an area of cool-looking amphibolite.
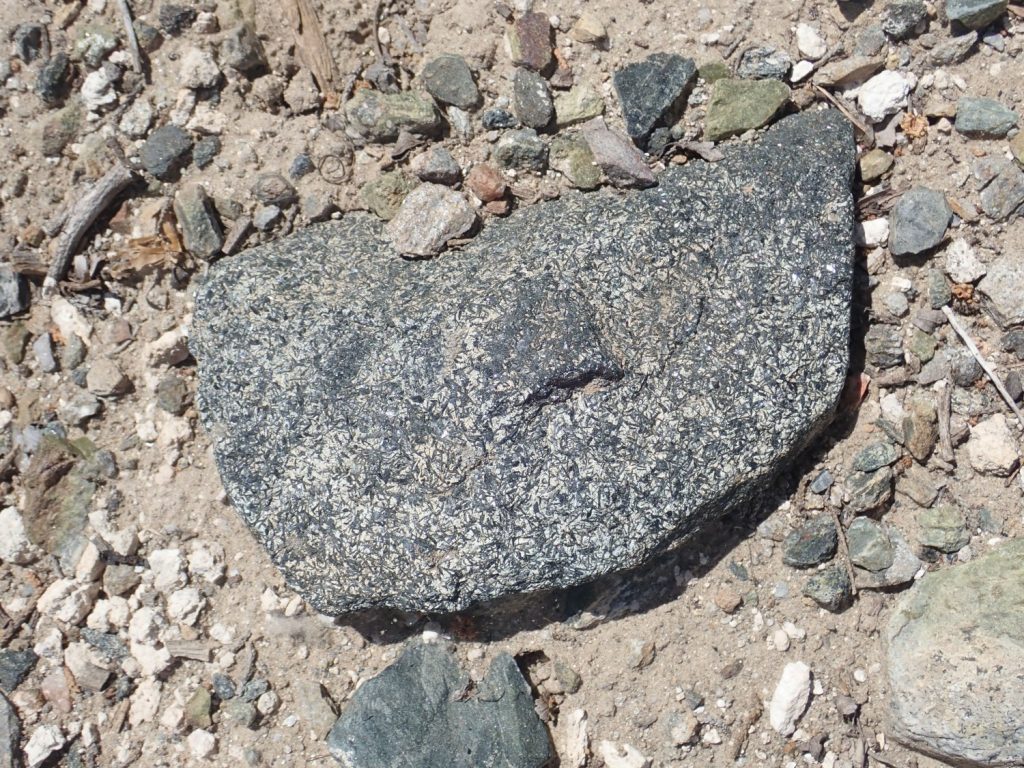
Those look almost like tourmaline crystals, a boron silicate. I have a very nice sample of tourmaline from my previous visit here. However, these might just be well-crystallized amphibole, and I leave it.
I get back to the mine. It’s now swarming with college kids. I like college kids, but they’re going to rapidly pick the place clean, so I decide I’m done for the way. One last stop on the way out:
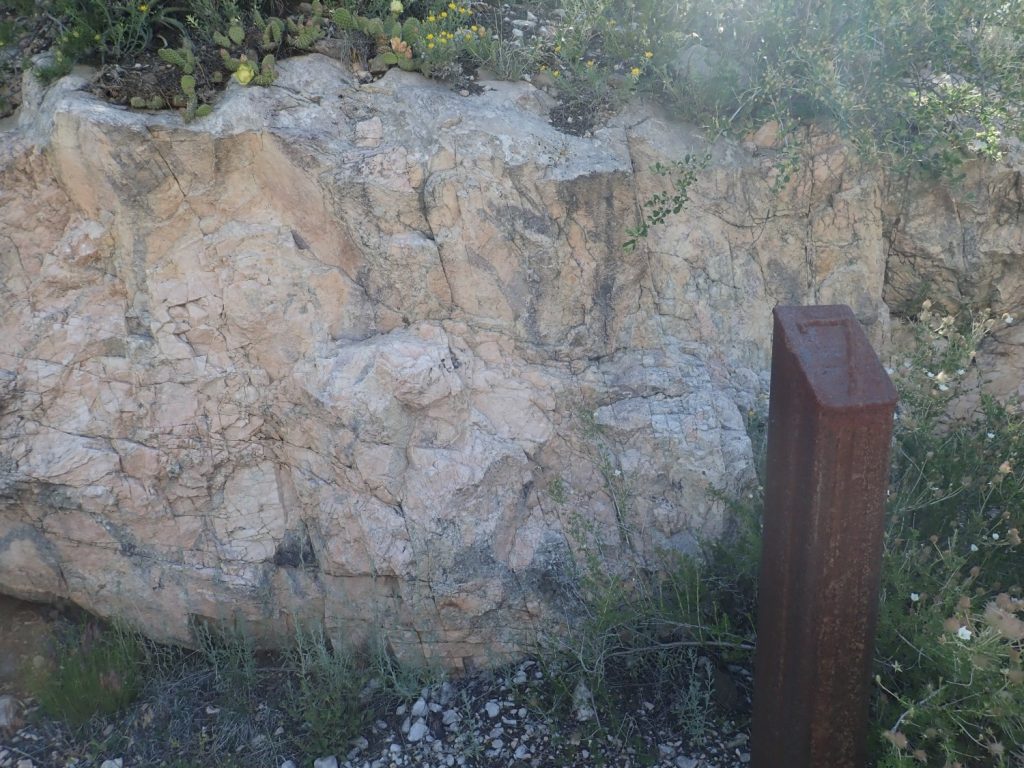
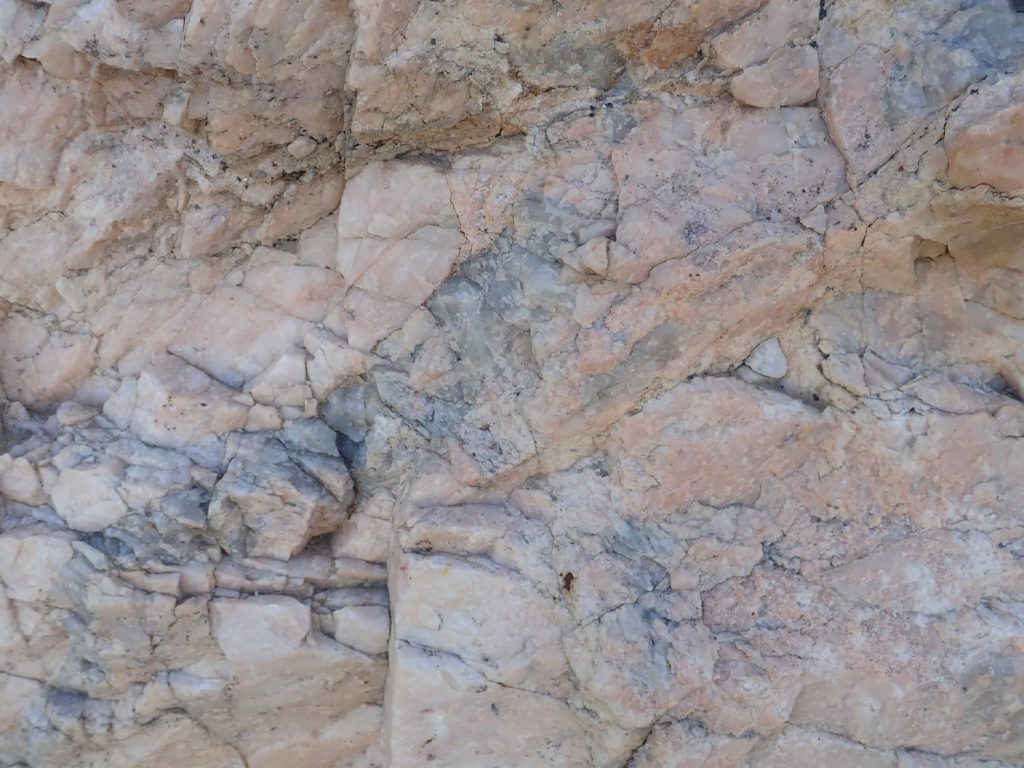
This is the aplite zone, the very bottom zone of the dike. It’s relatively fine-grained albite and quartz, according to the pamphet.
I hike back to the car and head on out. But a series of road cuts west of Dixon catch my attention.
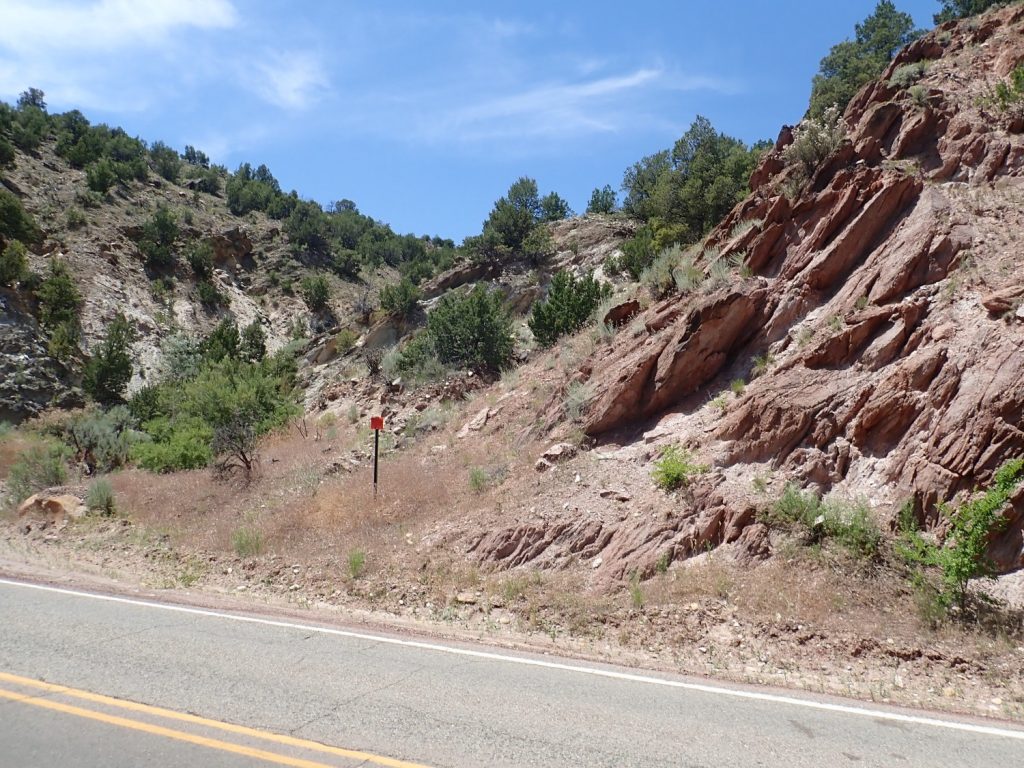
I’ve been driving past light grey mica schist when the schist abruptly turns a darker red. Intrigued, I pull over and explore.
Here’s where the color changes.
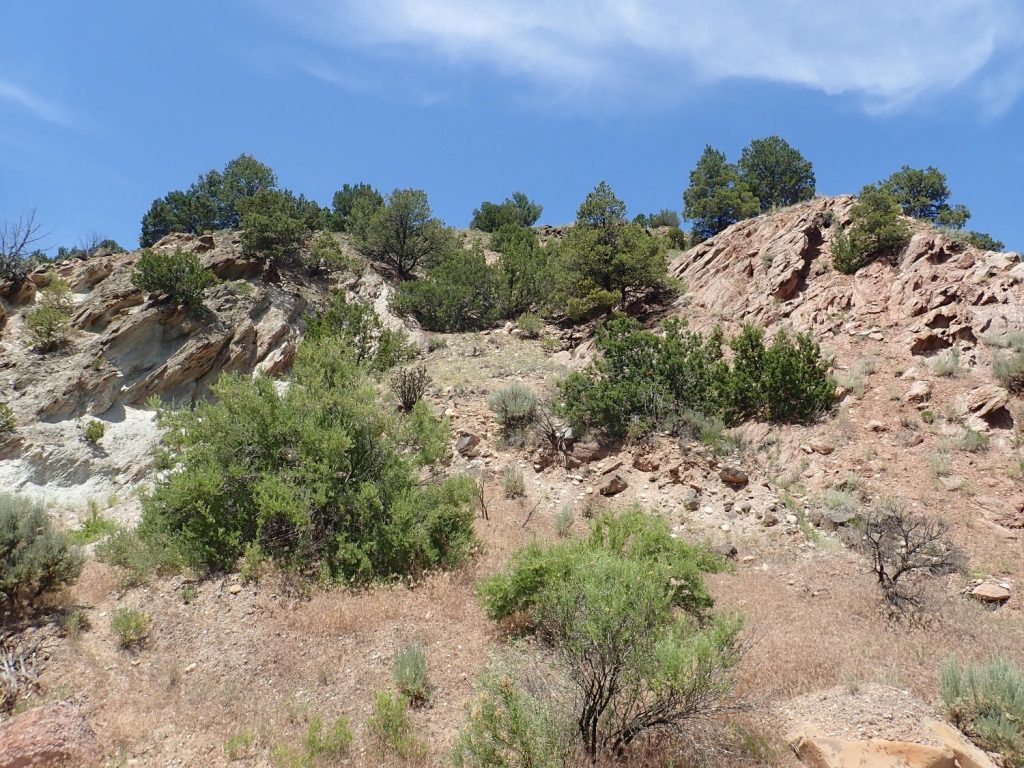
Here’s the schist east of the transition.
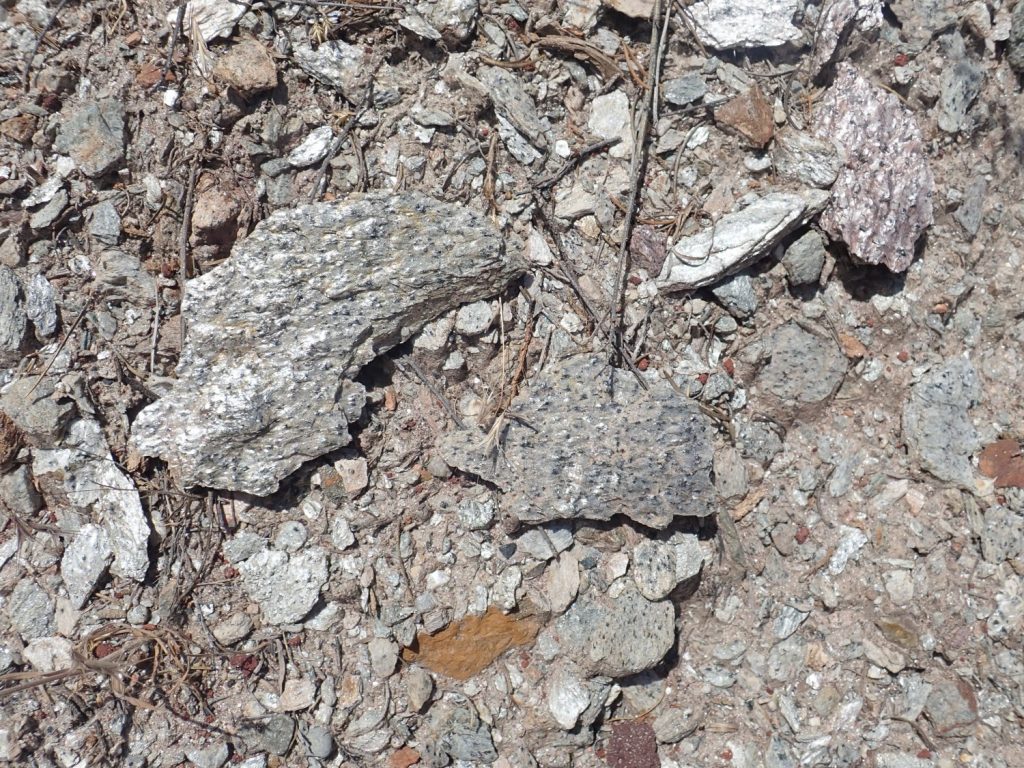
West of the transition.
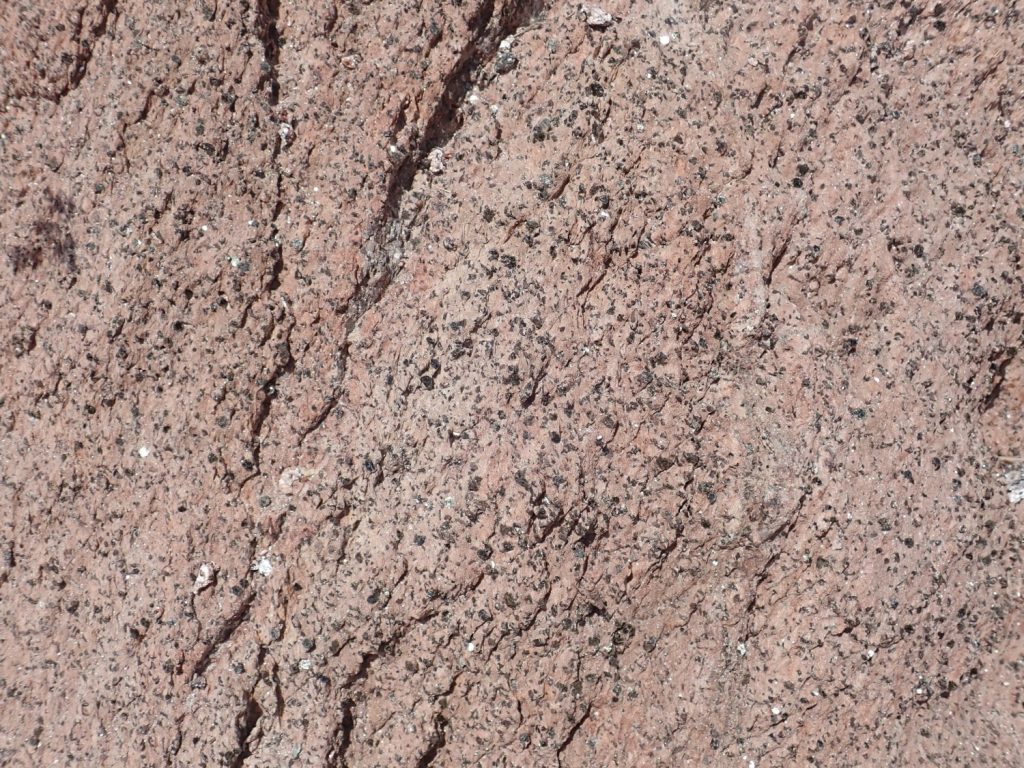
The only difference is the pink color. It reminds me of the pink manganese-rich zone just below the Ortega Quartzite in the top part of the Glenwoody Formation near Pilar, but this looks like Rinconada Formation, with all the black bits of staurolite.
As with the Pilar beds, there seems to be a ductile shear zone:
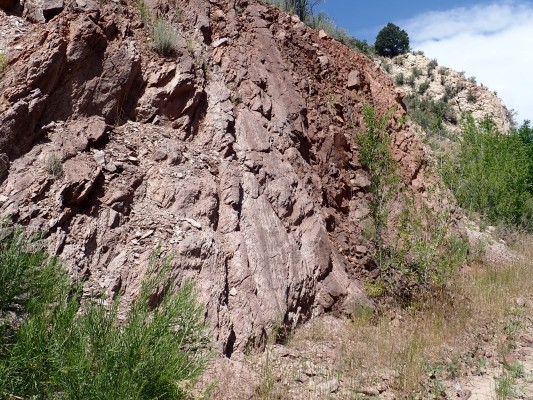
and then a zone of pink quartzite
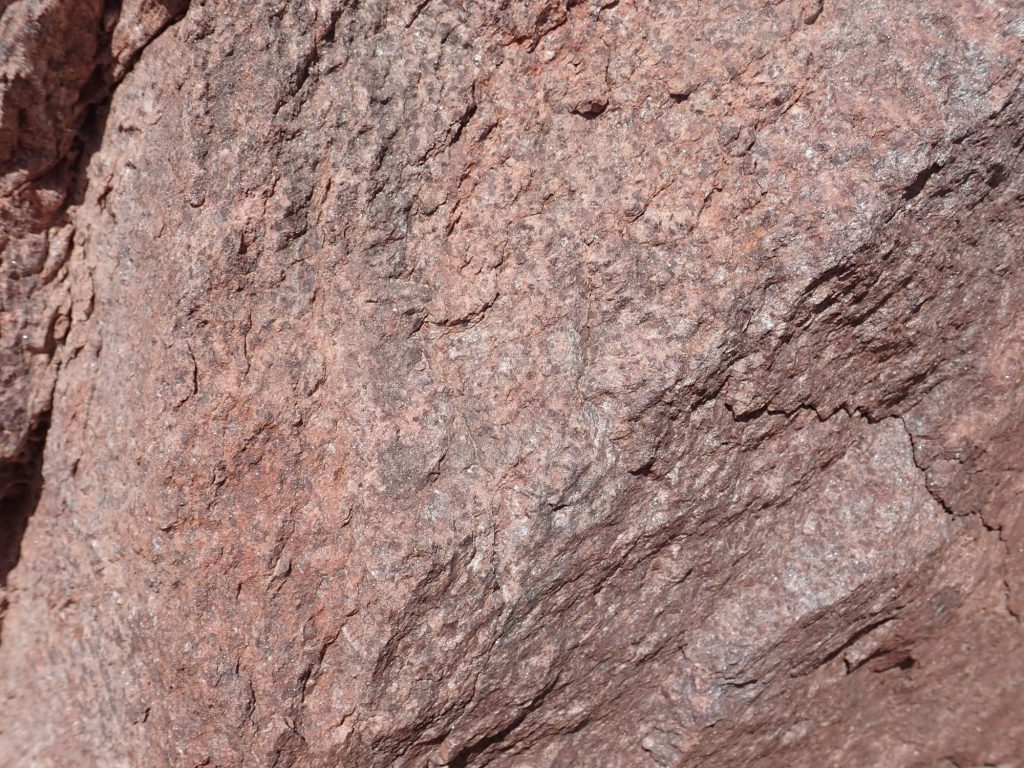
which transitions to light quartzite.
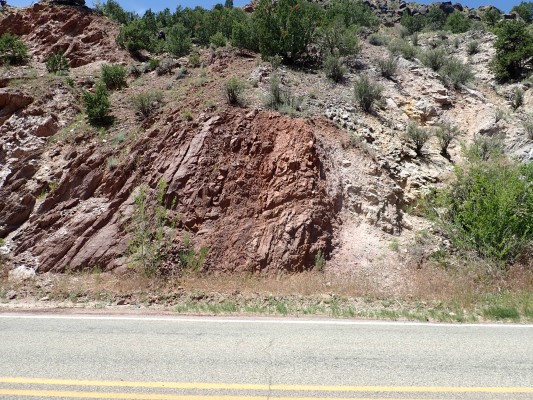
Here’s the thing. This looks an awful lot like the ductile shear zone at Pilar. The rock to the right looks very much like Ortega Quartzite,and is so identified on a geologic map of the Picuris Mountains. The rock on the left looks like Rinconada Formation, and is so mapped. But the Rinconada Formation is thought to be younger than the Ortega Quartzite. At Pilar, the shear zone is between the Ortega Quartzite and the older Glenwoody Formation, which is not a staurolite-bearing formation Go figure.
In any case, the ductile shear zone here is pretty cool.
I head for Santa Fe rather than home. There is an awful racket coming from the Wandermobile’s underbody every time the road is even a little bumpy, and I want to have it looked at. Along the way, a couple of photos for the book.
I’ve photographed this road cut in the Pojoaque Member, Tesuque Formation, before, but the light is much better now.
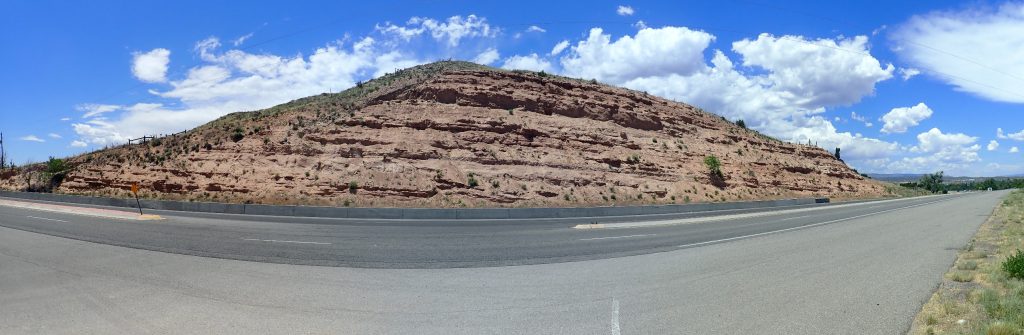
Rift fill sediments of the Rio Grande Rift.
And this is artsy as well as useful for the book:
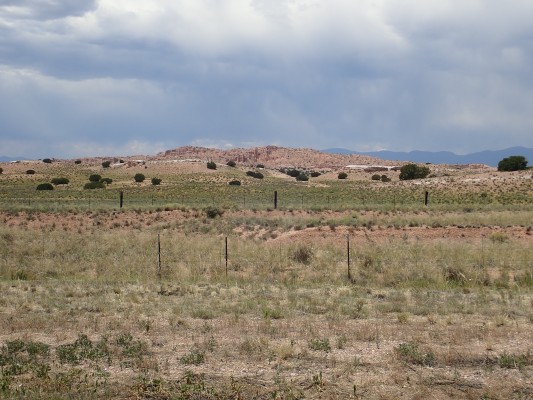
The light beds in the middle distance, capping the low hills, are the Upper 285 Road Ash, a volcanic ash bed 15.1 million years old — about the time the earliest Jemez eruptions would have taken place, though the vents are now buried and we can’t be certain this is from the Jemez. They cap Lithosome A of the Skull Ridge Member of the Tesuque Formation, Santa Fe Group, which is sediments eroded off the Sangre de Cristo into the Rio Grande Rift
The more distant hills are Los Barrancos, underlain here by Lithosome A of the Cuarteles Member of the Tesuque Formation, Santa Fe Group, eroded off the Sangre de Cristo slightly more recently, around 13 million years ago.
I go to Big-O; they won’t have a mechanic available to look at the car until Monday. I’m impatient; I go down the road to Jiffy-Lube. The look at it and determine that both my front shocks are shot and the front strut assemblies will need to be replaced: $1200. I kind of feared it was the shocks but I didn’t expect the repair to cost so much. But what can you do? I also get a betta fish for my small tank; been thinking about it for a while. He settles right in.
From there, home to give Cindy the bad news.
Monday I take precious vacation time off work to get the repair done. It does indeed fix the problem, and the new struts have lifetime warranties. (Though I probably void the warranty if I drive into a ditch deep in the mountains.) And betta is also doing fine.
Pingback: Hospitality | Wanderlusting the Jemez