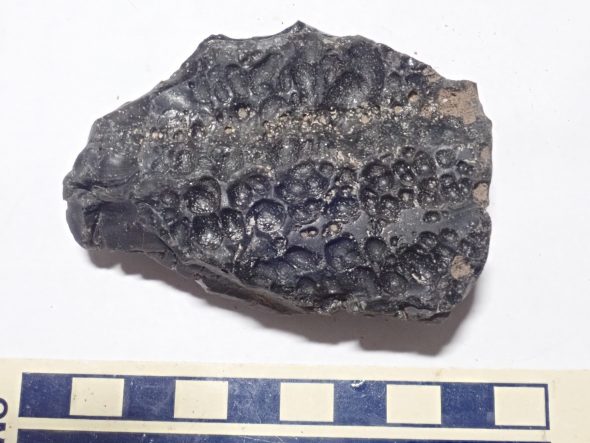
Wanderlusting obsidian
For some time, Gary Stradling has wanted to find some good chunks of obsidian on which to practice his knapping skills. (Not to be confused with “napping”, at which we are both already skilled.) Raw obsidian suitable for toolmaking must of course come in chunks large enough to work with, but the quality must also be adequate. Weapons-grade obsidian is uniform and free of inclusions or other flaws that would spoil the tools shaped from it. Such obsidian is not terribly common, but the Jemez Mountains have some good deposits, and Jemez obsidian (recognizable from its chemical signature) was traded prehistorically as far as the Ohio Valley.
There are a number of obsidian outcrops in the Jemez, but some are not of good quality (such as most of the Banco Bonito), or are located on private or protected land (such as the impressive prehistoric obsidian quarries of Cerro del Medio, now protected with the Valles Preserve), or have been picked clean. However, there are still some good exposures of high-quality obsidian not yet picked clean in the Forest Service areas south and east of Graduation Flats, the popular camping area so named for its traditional role in the graduation bacchanalia of Los Alamos High School.
Gary had his son, Jonathan, visiting with his family for the Fourth of July and this seemed like something his young grandchildren might enjoy. I, in turn, was eager to see some of the geology of the glowing avalanche deposits from Rabbit Mountain. So we loaded up Friday morning and headed out in a pair of vehicles. Our destination was a likely-sounding location called Obsidian Ridge, but I also had a backup plan (which turned out to be fortunate.)
We’ll get to the pictures in a moment, but first some background. Obsidian is volcanic glass, formed by a natural version of the process of glassmaking. The main ingredient is silicon dioxide, or silica, which is the chemical substance from which the common mineral, quartz, is made. Quartz sand of high purity is an essential ingredient for high-quality glass. This is combined with metal oxides, particularly alkali metal oxides such as sodium oxide or potassium oxide, to lower its melting temperature. The mixture is heated to its melting point and becomes an extremely viscous fluid that can be easily shaped. It is then quickly cooled, which prevents the glass from devitrifying, that is, from forming crystals. Glass is essentially a supercooled liquid at the molecular level. It’s a tangle of chains and clumps of silicon dioxide polymer broken up by the alkali metal ions.
Nature’s version starts with a high-silica magma (molten rock) that has a composition by weight typical of the volcanic rock known as rhyolite:
SiO2 73.2%
TiO2 0.2%
Al2O3 14.0%
Fe2O3 0.6%
FeO 1.7%
MgO 0.4%
CaO 1.3%
Na2O 4.5%
K2O 4.1%
This differs from typical window glass in having a lot less sodium and calcium and a lot more aluminum and potassium. Potassium is more expensive than sodium but makes little difference in properties, while aluminum makes for a higher melting point than calcium. But the chief difference is that glass makers take pains to exclude iron from window glass, because it tints the glass yellow to nearly black. Nature isn’t concerned with that, and so obsidian is almost always a deep black color.
Rapid cooling is another ingredient in obsidian. Magma often contains a great deal of water, which helps lower its melting point and makes it less viscous; otherwise, rhyolitic magma would barely make it out of the ground. However, water content would spoil the obsidian by allowing it to devitrify. So it is not enough that there is a supply of rhyolitic magma; it must retain its water long enough to approach the surface,then be degassed of its water content, then rapidly cooled.
This took place at Rabbit Mountain from a happy combination of circumstances. Rhyolitic magma was plentiful; there was a magma chamber full of the stuff ten miles across and a mile thick some ten miles under the surface 1.62 million years ago. This erupted as the Toledo Event, an enormous eruption that spread pyroclastic flows for miles in all directions and produced the Toledo caldera. This was a depression in the Earth’s surface that nearly coincided with the later Valles caldera we see in the central Jemez today.
The Toledo eruption degassed much of the magma that remained in the chamber, which was also hotter (and therefore less viscous) than the magma that was erupted in the Toledo eruption. The caldera was surrounded by a ring fracture where a plug of rock ten miles across dropped a mile into the partially emptied magma chamber to produce the caldera. The ring fracture was an easy path for the remaining magma to make it to the surface, and this erupted as domes of high-silica magma. Such a dome formed in Mout St. Helens after its 1980 eruption. But Mount St. Helens was puny compared with the Toledo eruption, and the latter produced many domes, mostly in the so-called Toledo Embayment. But two domes formed on the southeast side of the ring fracture, a small dome at Paso Del Norte, and a much larger dome as Rabbit Mountain.
The magma forming Rabbit Mountain was high in silica but mostly degassed, so it was ideal material for forming obsidian. Some obsidian can still be found on Rabbit Mountain today (though it should not be collected on the north side of the mountain, which is on the Valles Preserve.) However, this is mostly small bits of obsidian weathered out of devitrified beds. Here most of the obsidian absorbed enough water to crystallize and be spoiled as volcanic glass.
Only a small remnant of the Rabbit Mountain dome remains today. Most of the rest collapsed into the Valles caldera when it formed 1.25 million years ago. But a sizable part of the mountain collapsed when the dome was still forming, 1.43 million years ago. The red-hot and partially molten debris went roaring off to the southeast as a glowing avalanche, to form a deposit three miles long. The debris included enough new ash formed from the still-molten parts of the dome to cement everything together, but the rapid cooling of the flow preserved much of its obsidian content, which still remains pristine today.
The obsidian flow was later buried by the Tsherige Member of the Bandelier Tuff, the great ash flow formation of the Valles Event. Where this has been eroded through, the obsidian flow is exposed beneath. Obsidian Ridge is such a location, where the finger mesa between Frijoles Canyon and Alamo Canyon has been eroded to a knife edge.
I have been on the access road exactly once before, and only a short distance (hiking), so I was not sure what to expect. The access road:
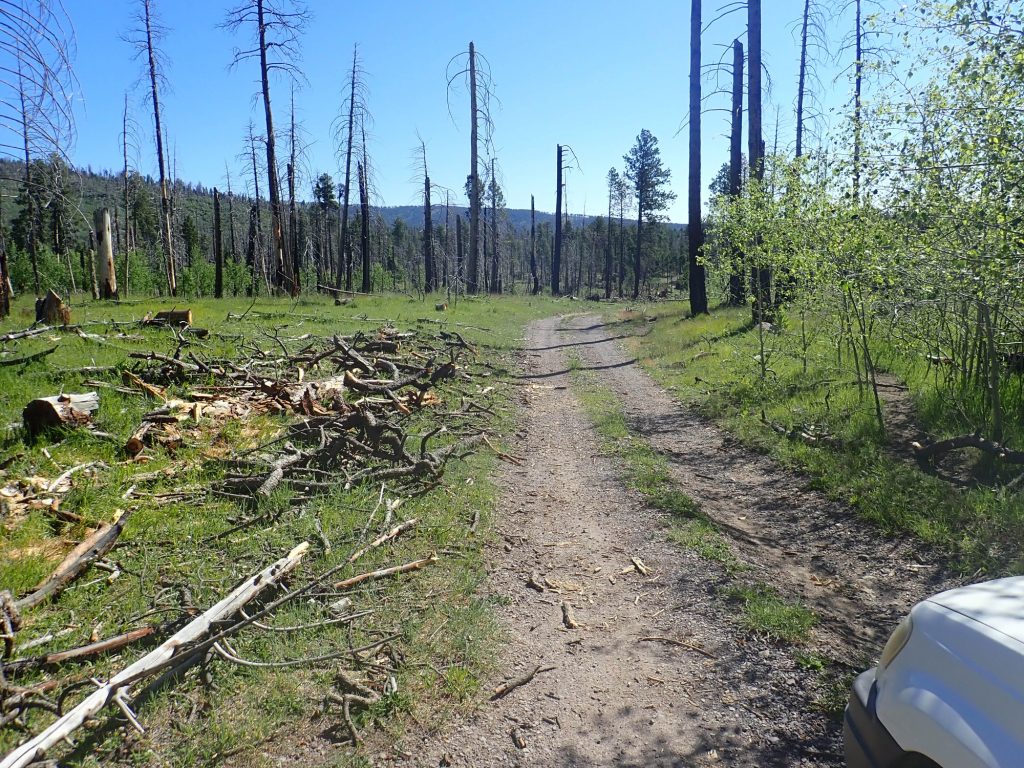
Although Graduation Flats itself was mostly spared by the Las Conchas fire, the fire devastated most of the surrounding area. I was with Cindy and some Cub Scouts on my one previous visit; she’ll be sad to see the extent of the damage.
This immediate area is underlain by a small landslide off Rabbit Mountain that followed the Valles eruption and so is located on top of the Tsherige Member. The mountain had long cooled by then and this flow does not contain ash and is not sintered together. It contains some small bits of obsidian — hence my hike with Cindy and the Cub Scouts years ago — but not the big weapons-grade chunks. I saw practically none here; we’re close enough to Graduation Flats that this area has probably been picked clean.
Further down the road, a big disappointment.
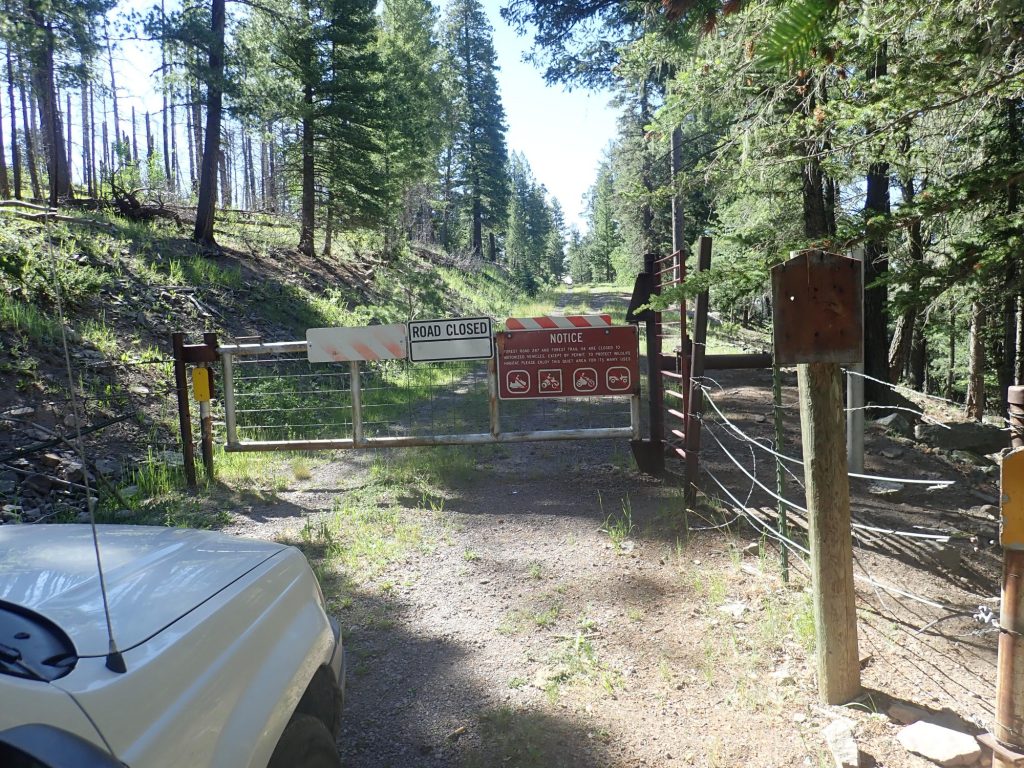
The road is closed to motor vehicles. We debate. The road is open to hikers, but I estimate it’s about two or three miles to Obsidian Ridge. (Almost exactly two miles, when I measure it on Google Maps later.) That’s too much for young children. We go with Plan B, which fortunately turns out to be a rousing success.
Plan B is another exposure of the obsidian beds, along Dome Road. Here the road passes a knoll of much older Paliza Canyon Formation dacite, around which the glowing avalanche swept and ponded. The later Tsherige Formation pyroclastic flows probably never covered this area, finding lower ground to the west and east, and so the obsidian flow was preserved and is exposed at the surface today. In fact, the parking area is thick with small bits of obsidian, which Gary’s grandkids are delighted to collect at once.
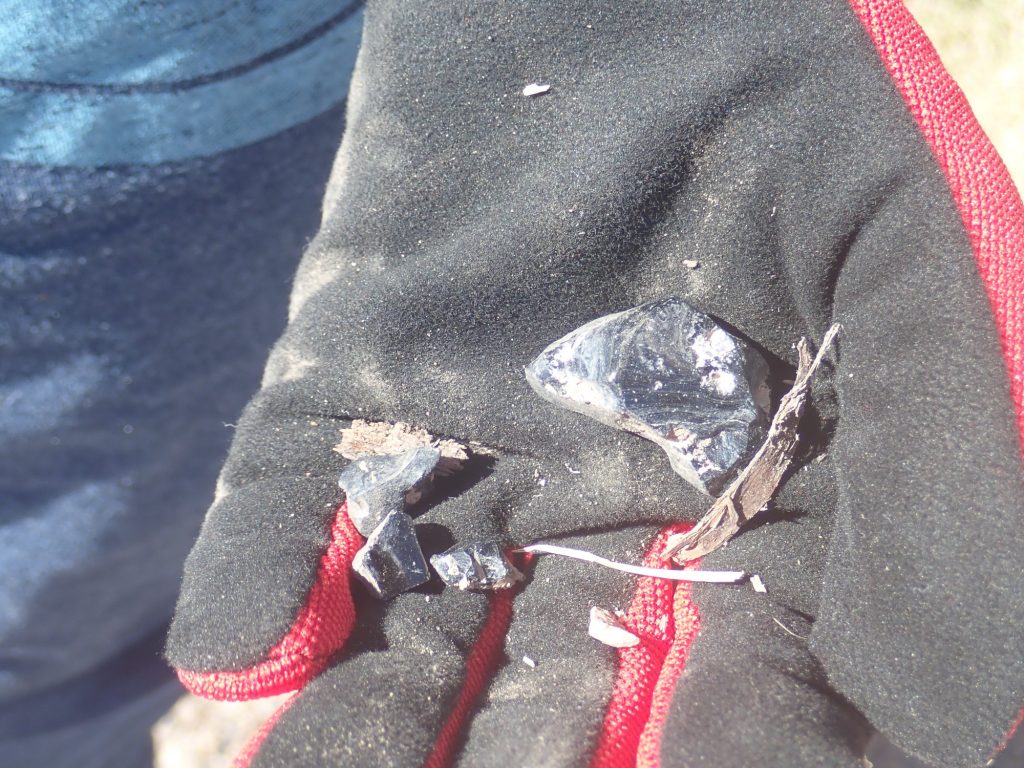
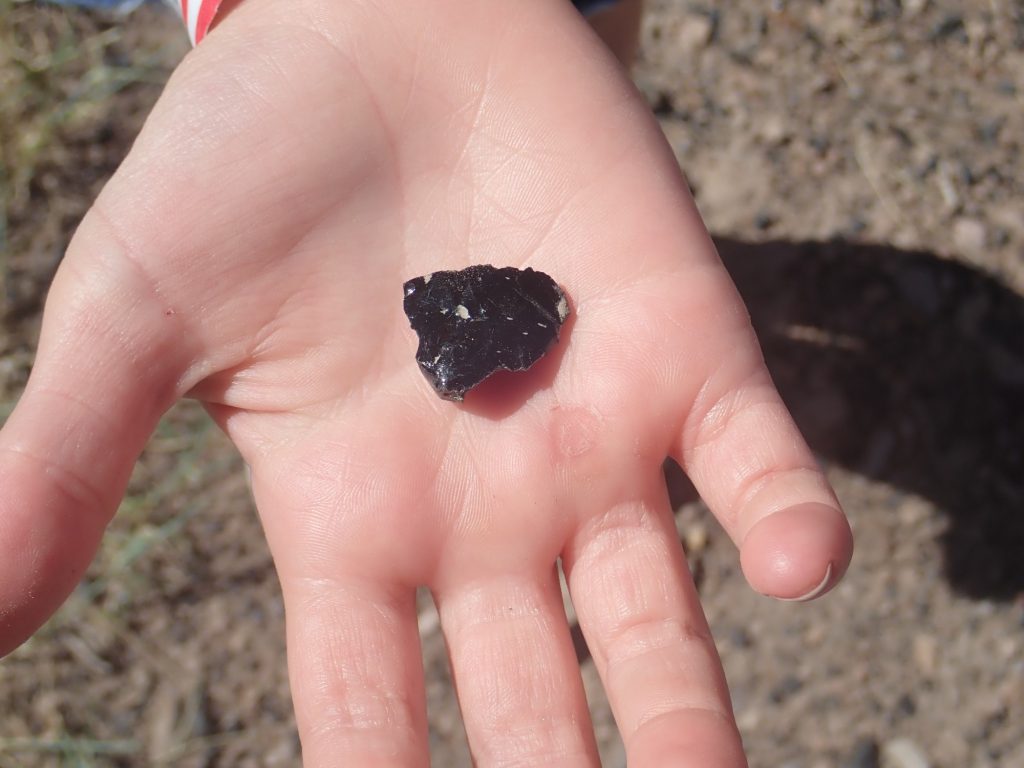
The best hunting is to the east, just across the road, where chunks of high-quality obsidian a few inches across are abundant. Gary soon is collecting obsidian around a fallen tree.
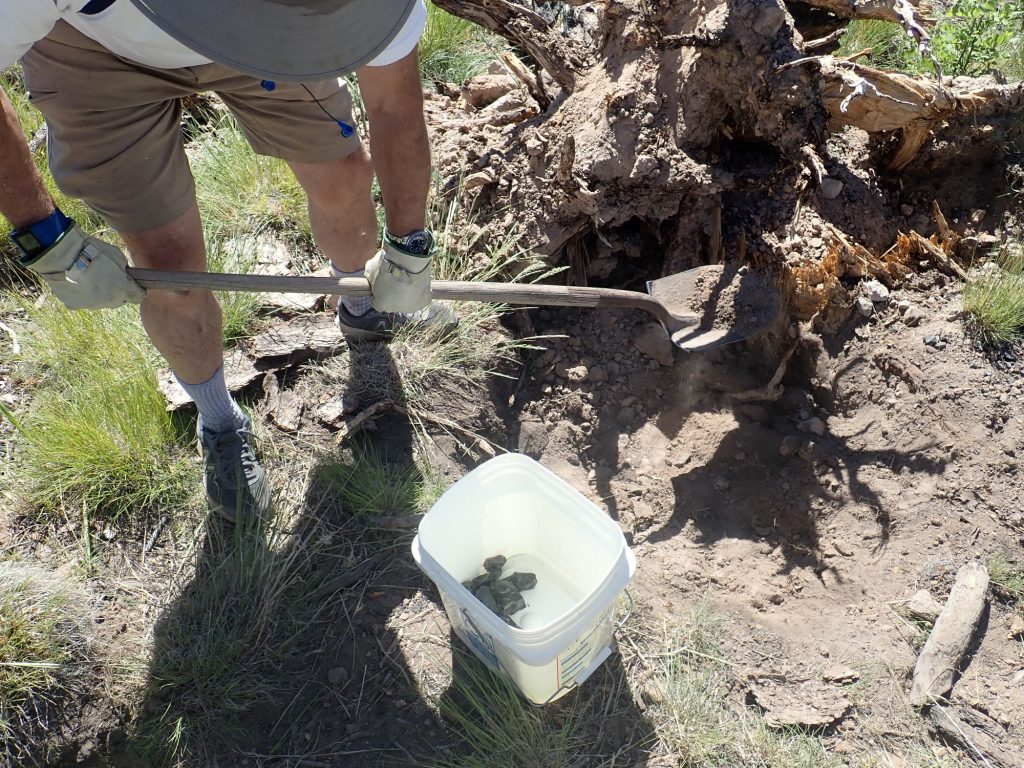
The glowing avalanche, while rich in obsidian, was still mostly devitrified obsidian that is an unremarkable gray rock usually identified as rhyolite.
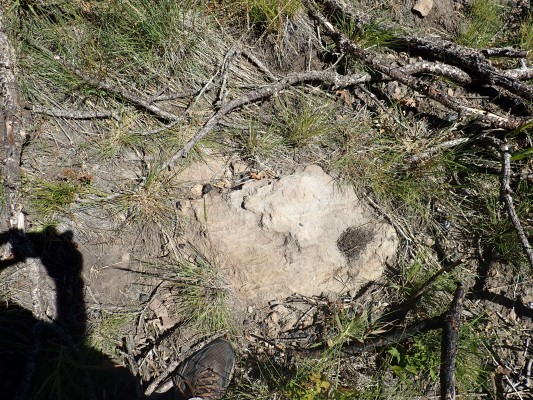
There’s a small weather station up here, next to a fenced area of very small pine trees.
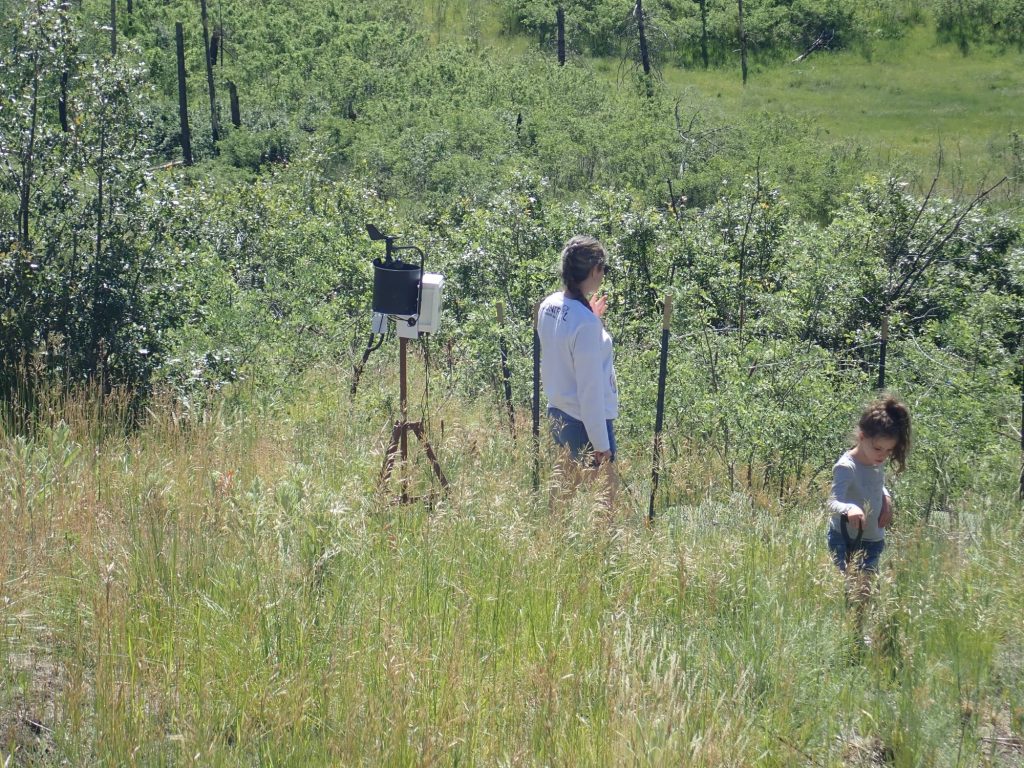
The ones here are doing okay. Others we come across are not surviving well, which is too bad. The Forest Service is doing its best to reforest the area but the fire was devastating enough that full recovery may take centuries.
I get some good obsidian samples, as many as I want. This obsidian shows flow marks.
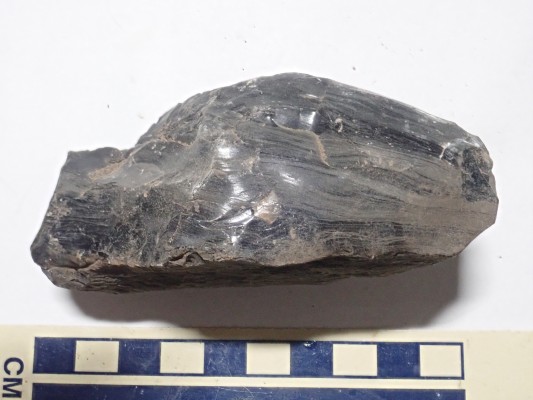
Perhaps not ideal for tools, due to the nonuniformity, but still pretty high grade.
This sample shows numerous tiny spherulites:
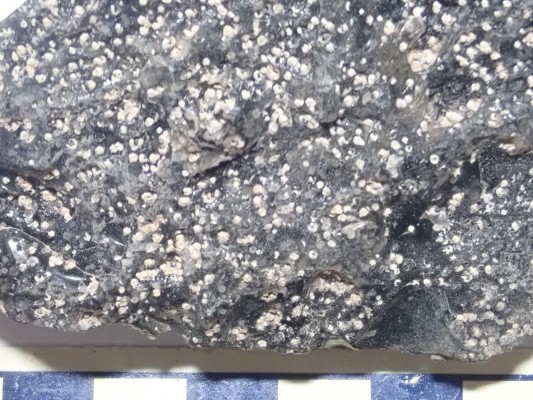
Spherulites are gas bubbles in the original magma that become filled with crystals as the obsidian cools. They spoil it for tool use, but enhance its ornamental value. Here’s a sample where there were likely once large spherulites that have weathered out.
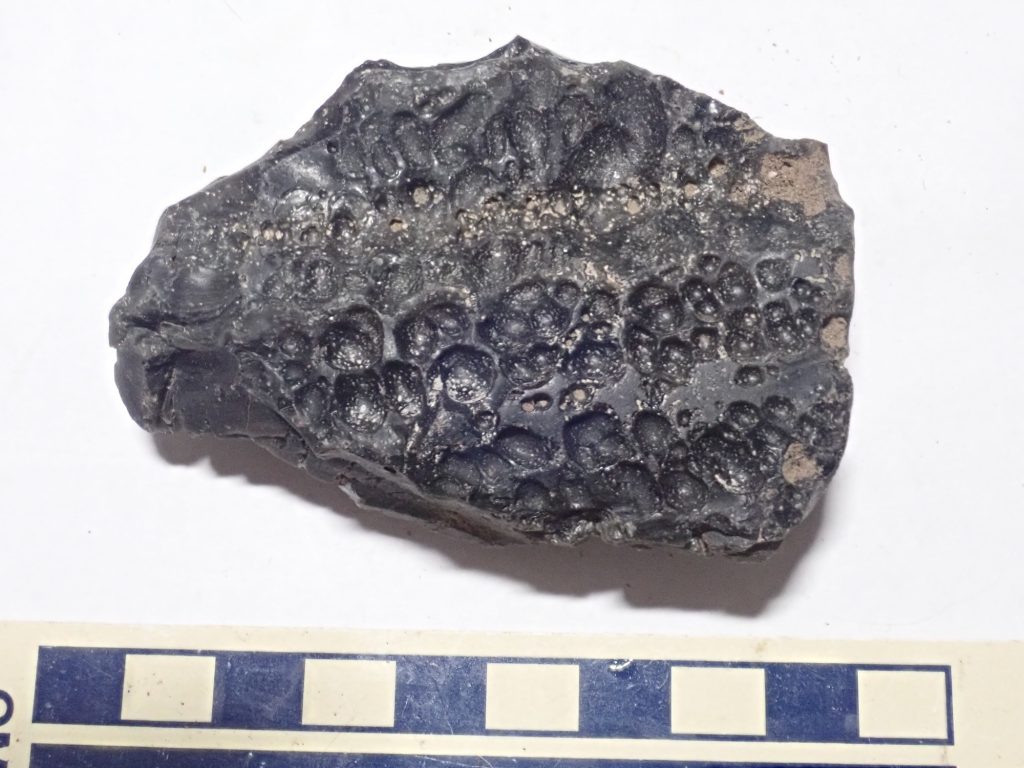
This one likely was from the top of the flow, and shows both spherulites and cracks from too-rapid cooling.
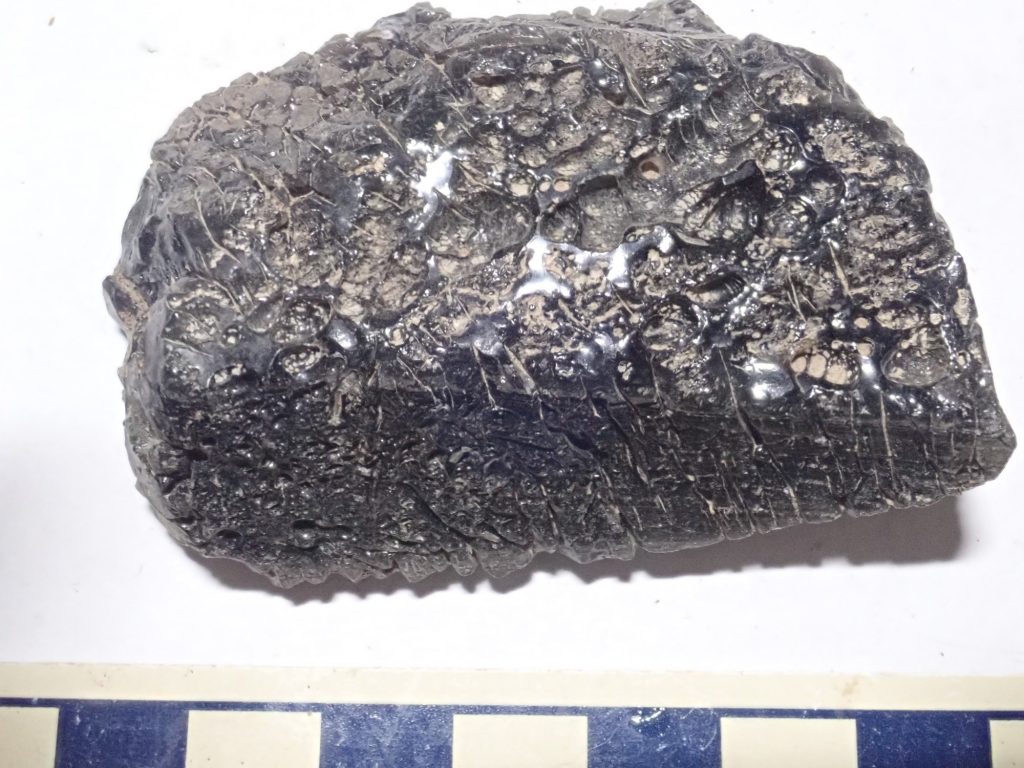
This sample is mostly devitrified, but with a few ribbons of obsidian remaining.
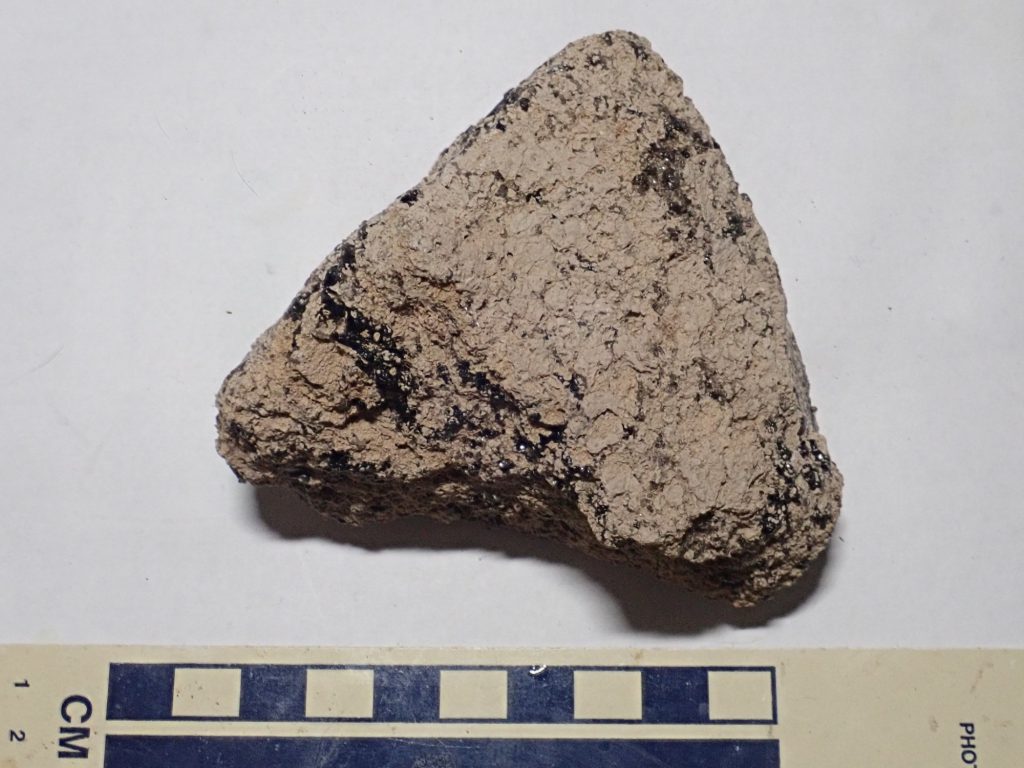
I then explore the area. Gary suggests quite sensibly that obsidian may have accumulated in the small valley to the east, but as I descend, I find that the best obsidian was atop the flats. There is another moderately rich layer about twenty feet down section, but beyond that, there is no more obsidian. Too bad; it was a good suggestion.
There is also a scattering of El Cajete Pumice over the area.
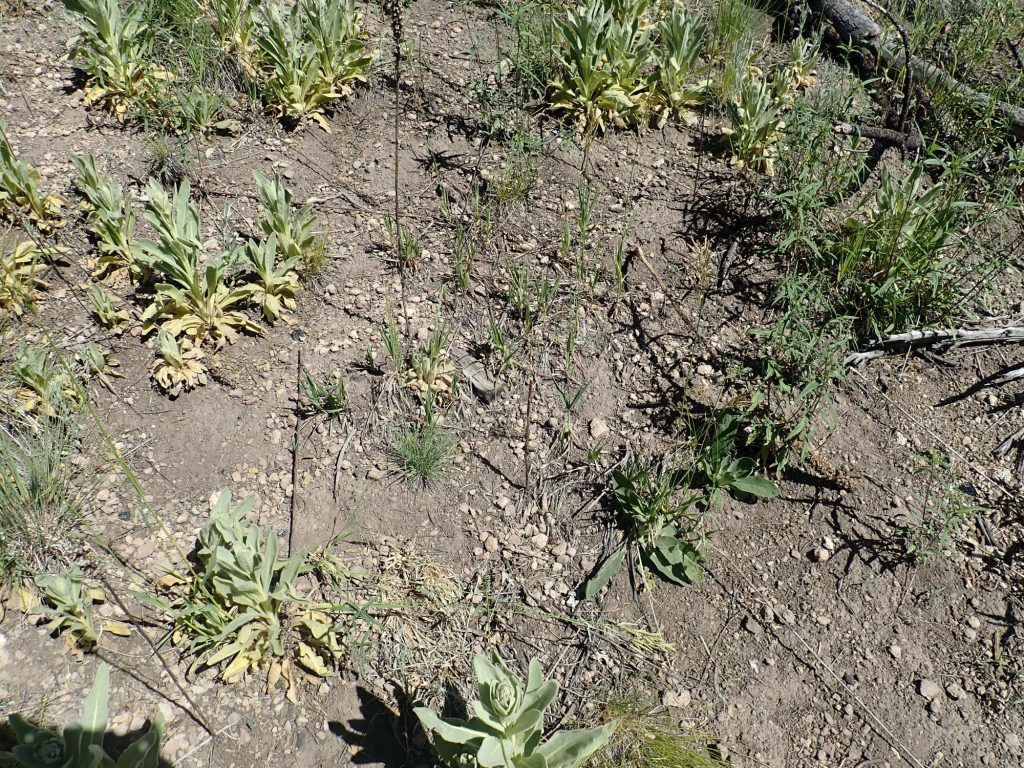
The El Cajete Pumice is one of the youngest formations in the Jemez, so much so that its age was long highly uncertain. It falls into an awkward age range that is too young for the usual radiometric measures (no time for the daughter products to build up) and too old for things like carbon-14 dating. However, extremely high-precision Ar-Ar dating by the new radiometric laboratory at New Mexico Tech has finally produced an age of 73,000 years that we can have some confidence in. That’s practically yesterday in geologic terms: The Jemez Mountains are still a “live” volcanic field, though sleeping at the moment and unlikely to erupt again in our lifetimes.
El Cajete Pumice blanketed much of the southern Jemez, and deposits can be found as far east as the Cerros del Rio. You occasionally find it in the canyons south of White Rock. It can be distinguished not only for the fact that it’s on top of older beds, but by its tan color, from its unusually high iron content. It sometimes contains visible needles of hornblende and biotite mica, though I didn’t immediately spot any in the samples here.
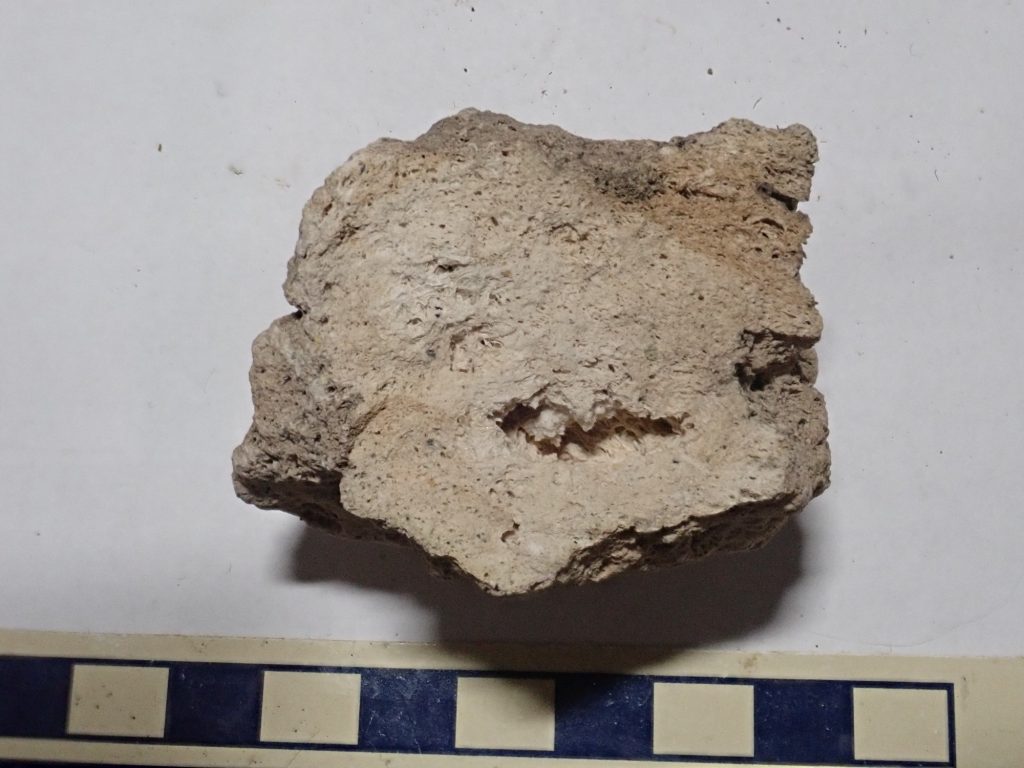
The dacite knoll to the north.
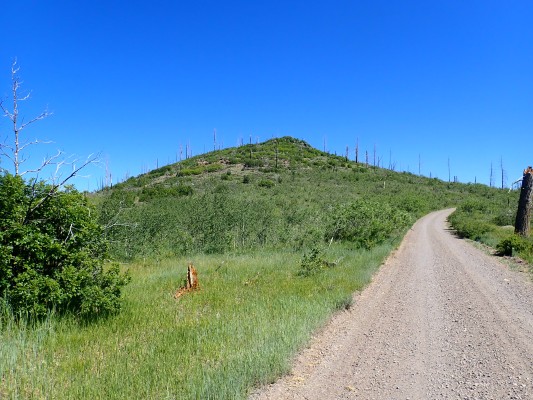
I think about climbing this, for its view from the top, but after a brief excursion on its lower slopes, I find it covered with thorny shrubs and decide it’s larger than it looks. (General bushwackingn rule: It’s always larger than it looks.) Time is limited so I climb the much smaller knoll just south of the parking area.
Both knolls are underlain by hornblende dacite.
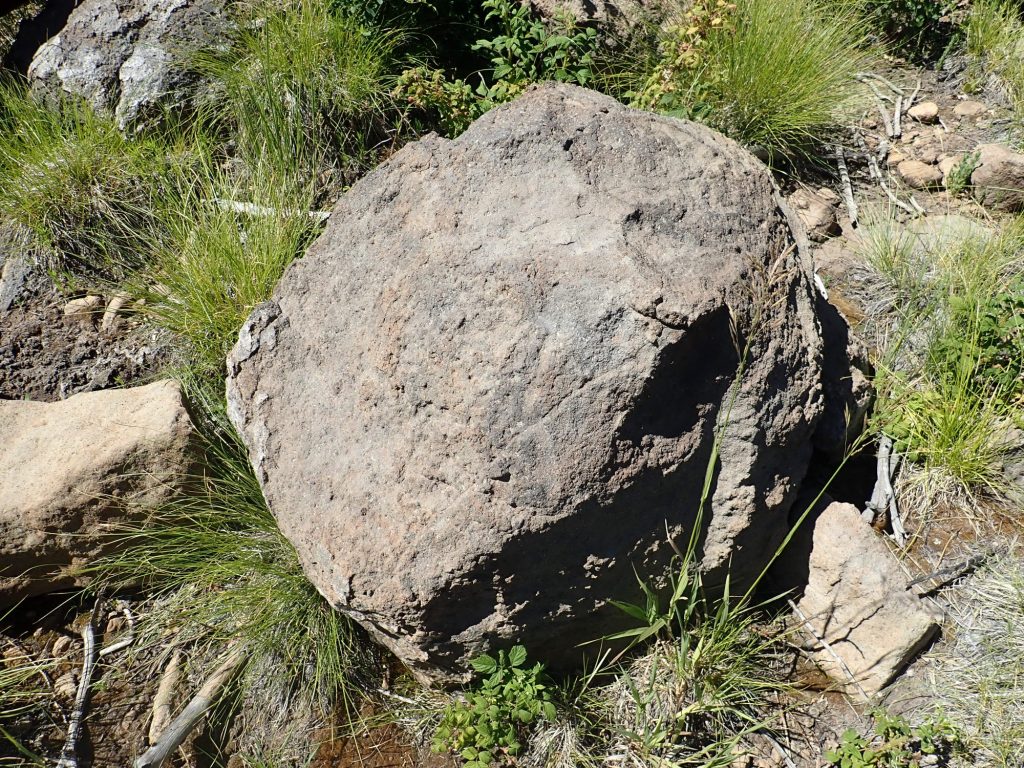
Dacite is similar to rhyolite but a touch poorer in silica and alkali oxides. (Implying more calcium, magnesium,and iron.)
From the top, I get a very nice view of Aspen Ridge to the west.
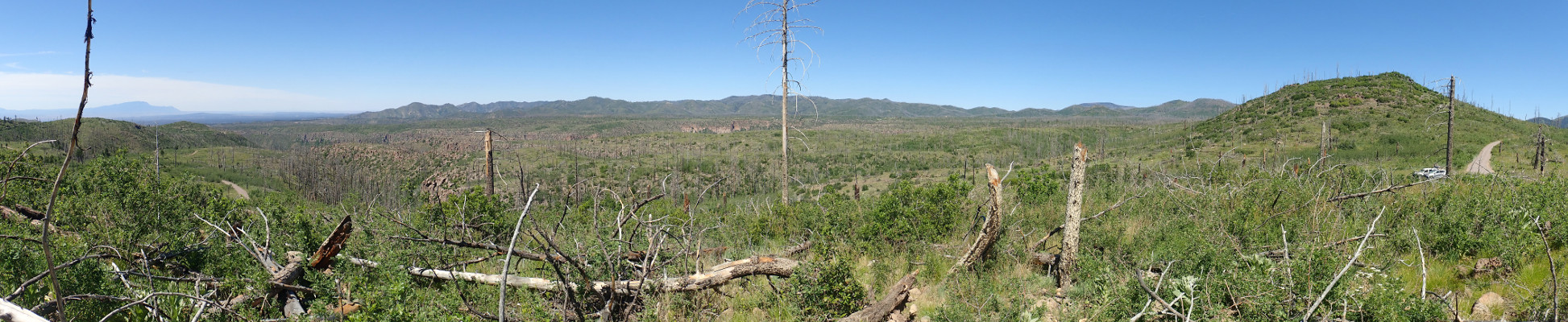
As with most images at this site, you can click to get the full-resolution version.
Aspen Ridge is the easternmost of a series of north-south ridges forming the Keres Highlands, the wild southern part of the Jemez Mountains. Like the knoll I’m standing on, it is underlain by various flows of the Paliza Canyon Formation, the largest formation of the Jemez Mountains. This formation accounts for better than half the original volume of the volcanic field and is mostly andesite, a volcanic rock of moderate silica content, with smaller amounts of more silica-poor basalt and more silica-rich dacite. This is intruded by plugs and dikes of the Bearhead Rhyolite, a very silica-rich rock named for Bearhead Peak at the south end of Aspen Ridge (at left in the photo.)
The area between my knoll and Aspen Ridge was low ground now covered by mesas of the Bandelier Tuff erupted out of hte calderas to the northwest. The originally uniform layer of tuff has been eroded into finger mesas by multiple canyons resembling those of the Los Alamos area.
Here’s a second panorama of the ridge at maximum zoom.

Beware; the full resolution image may take some time to load. However, it shows that the next ridge to the west, Peralta Ridge, is visible right of Bear Mountain, as is the high point of Peralta Ridge, Las Conchas, which peeks over Aspen Ridge at center right as a sharp peak.
After some discussion, we decide the kids have just enough attention span left to visit the Cochiti overlook. It takes me a minute to recognize this:
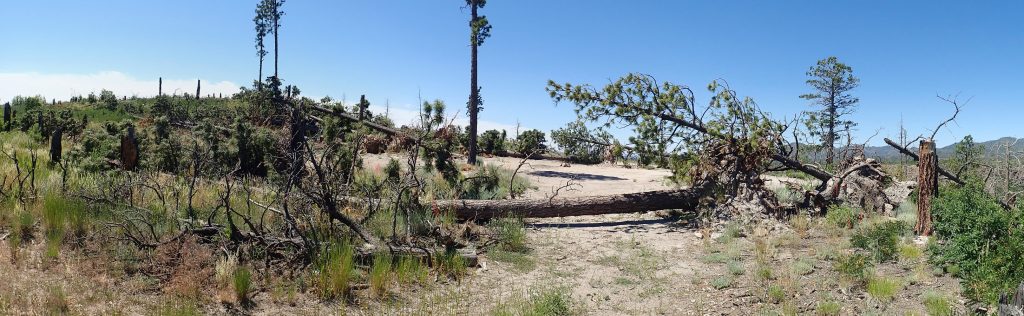
That’s spectacular wind damage from the “bomb cyclone” we had last winter, which brought sustained very high winds to our area at a time when the ground was already very soft from heavy precipitation. The overlook here had some of the few old ponderosa pines in this area that were spared by the fire, but the lack of nearby forest amplified wind force and brought these down. Sad.
Wildflowers:
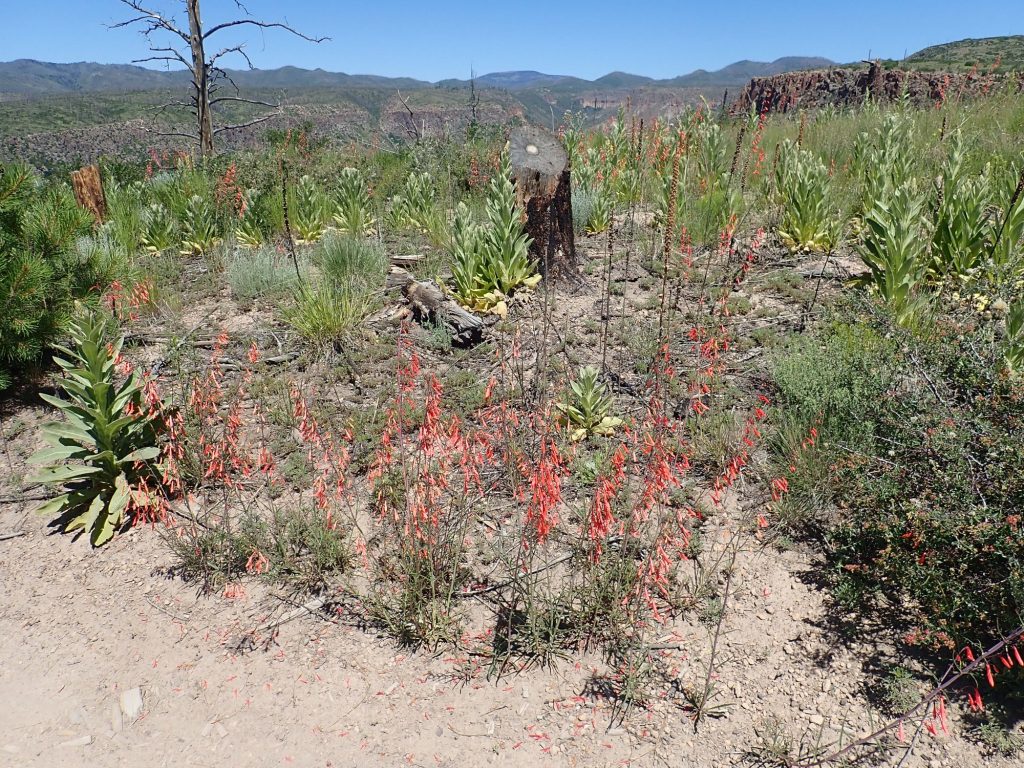
A species of red penstemon. There are several, but a good guess is Penstemon barbatus, common in the Four Corners states.
Of course, it’s called an overlook for a reason.
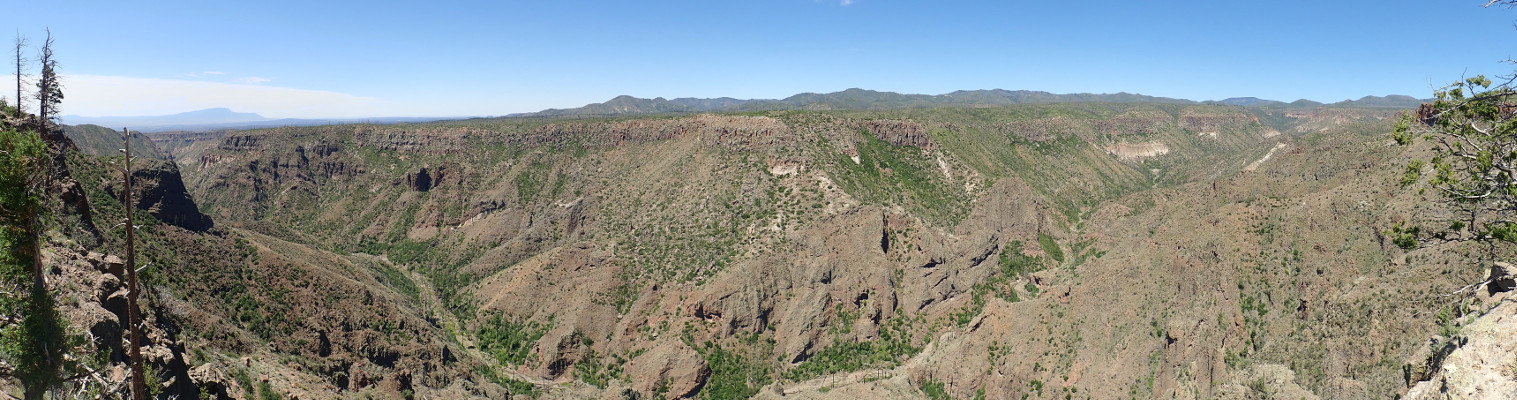
There are at least five units here. The lowermost dark irregular beds are volcaniclastics (broken rock produced by volcanic activity) of the Paliza Canyon Formation. These were probably erupted from the San Miguel Mountains just to our east here. There is a white dacite tuff bed halfway up the canyon at left that has been dated to around 9.1 to 9.4 million years old, typical of the Paliza Canyon Formation. Above is a second round of volcaniclastics. These were clearly eroded into rugged terrain before being buried under light beds of the Otowi Member of the Bandelier Tuff, erupted in the Toledo Event 1.62 million years ago. These beds are not everywhere well exposed, being covered in many places by colluvium (stuff that fell down from higher up.) Then comes the canyon rim, a final darker layer of Tsherige Member tuff from multiple pulses of the Valles Event, 1.25 million years ago.
At right are the best exposures of Otowi Member:
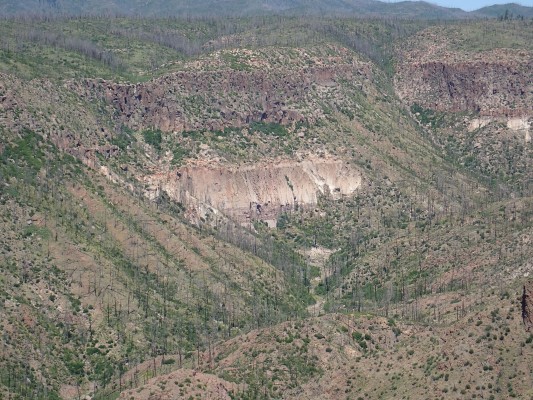
You can see a base layer just showing at the bottom of the canyon, which may be air fall pumice from the start of the eruption, then darker layers that may be either broken rock from the caldera collapse or surge beds from the beginning of the pyroclastic flows. I would love to get down there and get a closer look, but there’s no safe way in and

There was once a road in from the Dixon apple orchard, but that is utterly washed out. In any case, Cochiti Pueblo has closed the south route, as part of a land dispute with the state of New Mexico regarding the Dixon area. It might be possible to get in by hiking down the headwaters of Spruce Canyon near Graduation Flats, but the distance is 3.5 miles one way over rough terrain and the danger of flash floods is great. I won’t be getting to those beds for closer examination any time soon.
Still, we got some excellent obsidian, the kids seemed to have a great time, and I’d call the day a solid success.