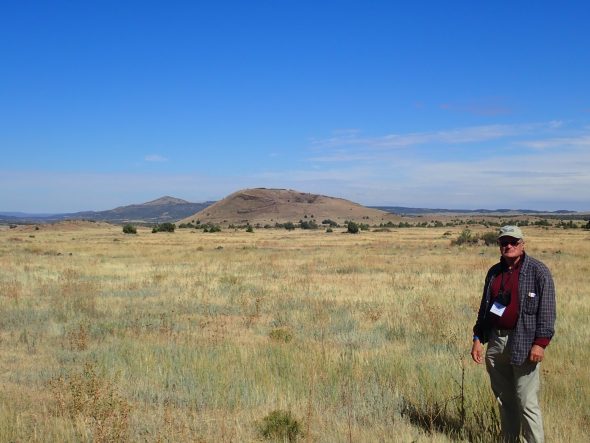
Wanderlusting Clayton, day 2
Gary and I wake to find it overcast and somewhat cool. The ground is wet enough to show we had rain during the night. However, the tent stayed dry inside and we enjoy a camp breakfast of bacon, eggs, pancakes, and steel-cut oats.
We make it to Clayton in time to board a conference van. The vans head out to Capulin as we make introductions. Our driver is Nels Iverson of the New Mexico Bureau of Geology, who I learn is studying extensional tectonics in Antarctica and Africa. It’s relevant to New Mexico, where the Rio Grande Rift is a product of such tectonics and is responsible for more than its share of the geologic hazards in the state.
Arriving at the Capulin visitor’s center, where the crowd gathers around the snack and drink tables.
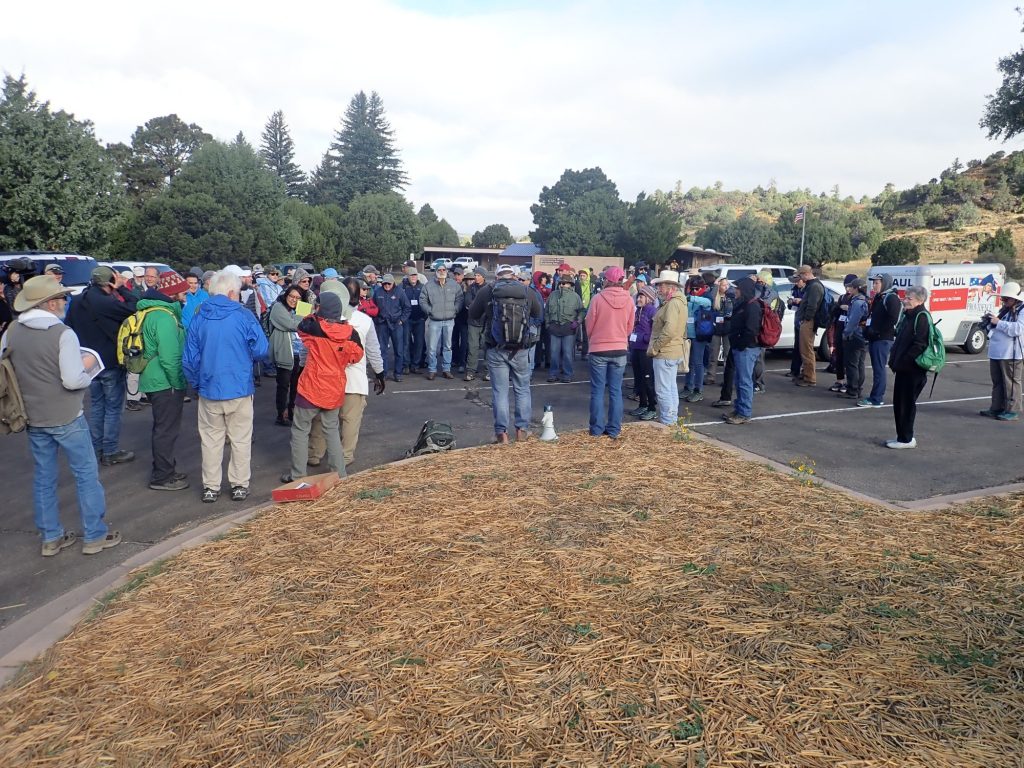
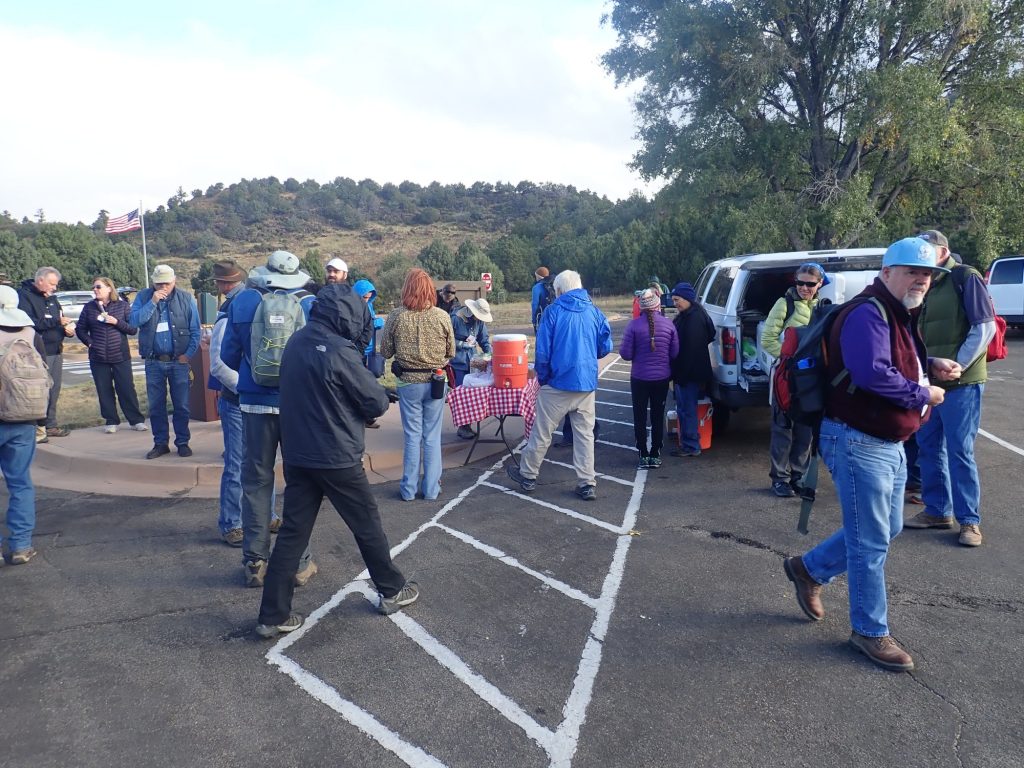
In the background is Capulin Mountain itself.
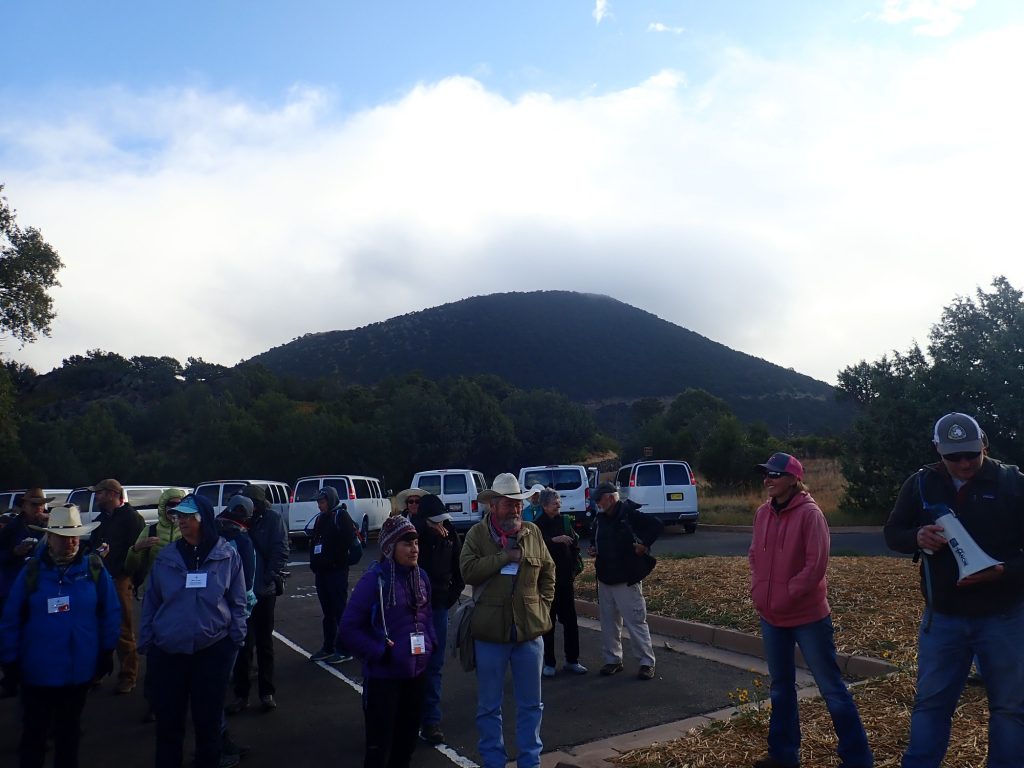
Unfortunately, the road to the summit has been spectacularly washed out by recent severe thunderstorms, and we will have to content ourselves with hiking around the base of the mountain. We begin by trooping off to the Sierra Grande viewpoint.
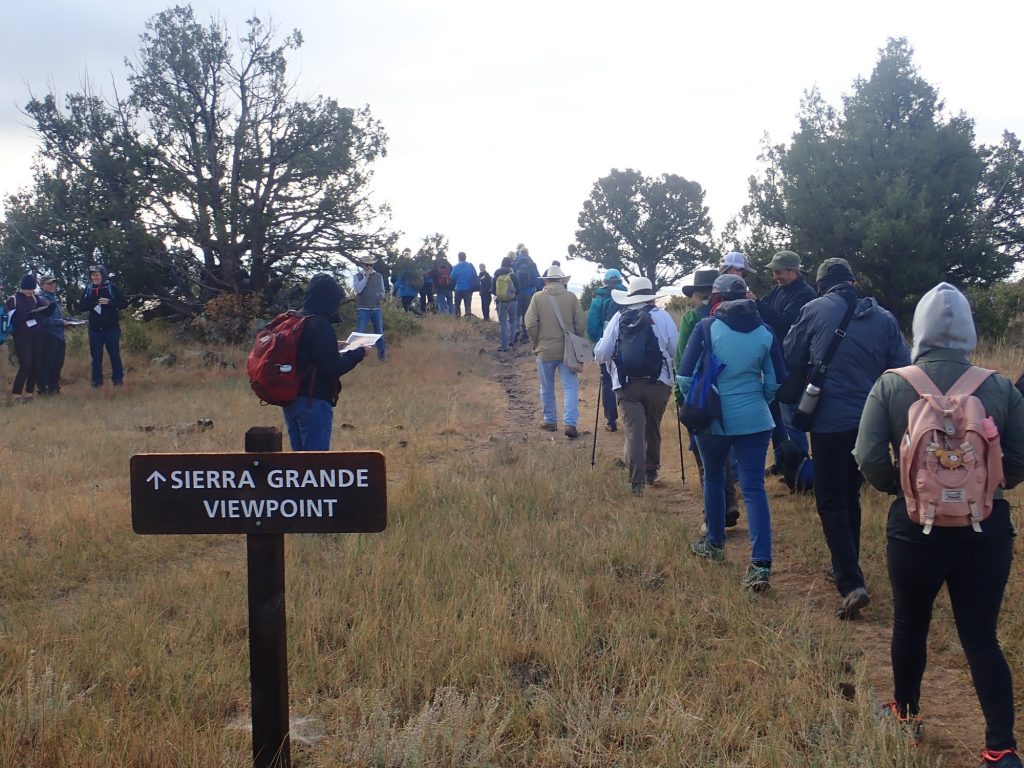
By amazing coincidence, the Sierra Grande Viewpoint gives an excellent view of Sierra Grande.
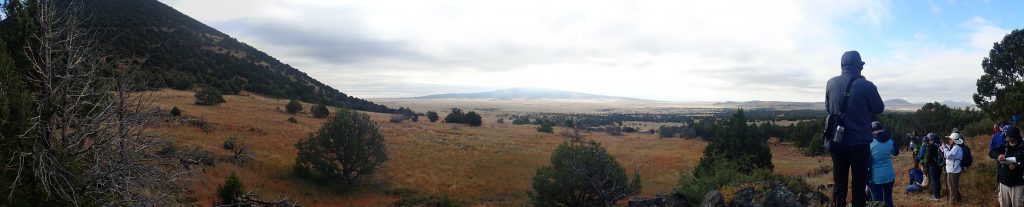
(Click to enlarge, as you can do with almost all images at this site.) Sierra Grande is the broad mountain in the distance at the center of the panorama. It is part of the Clayton phase of the Raton-Clayton volcanic field, which peaked around 3 to 2.25 million years ago. (I described these phases in the previous post.) This phase included the only significant andesitic eruption of the volcanic field — that of Sierra Grande itself, the largest volcano in the field, which is underlain by two-pyroxene andesite.
Let’s unpack that. Andesite is a volcanic rock with an intermediate content of silica, around 60% by weight. This contrasts with silica-poor basalt at 50% silica content and silica-rich rhyodacite at 70% silica content. Since silica makes lava viscous, basalt tends to be runny and form thin flows, while rhyodacite is extremely viscous and is extruded out of the ground as steep plugs and domes. Andesite, with its intermediate viscosity (about the same as smooth peanut butter), is viscous enough to form the classical cone-shaped volcanoes known as stratovolcanoes. Sierra Grande is thus the only stratovolcano in the Raton-Clayton field.
Pyroxene is a mineral common in low- to intermediate-silica volcanic rocks. It is composed of chains of silicon atoms surrounded by oxygen atoms, which are bound together like sticks in a bundle by iron, magnesium, and calcium atoms. Two-pyroxene andesite contains both calcium-rich pyroxene (clinopyroxene) and calcium-poor pyroxene (orthopyroxene). This is a very common kind of volcanic rock, which also makes up much of the Jemez Mountains back home.
At left is Capulin Mountain. This is a cinder cone erupted around 54,000 years ago; not quite the youngest volcano in the field, but close. It is part of the Capulin phase of the Raton-Clayton field. A cinder cone forms from low-viscosity magma with a fair amount of dissolved gas. The gas bubbles out of the magma as it approaches the surface, providing enough force to cause the magma to fountain hundreds of feet into the air. Much of the magma solidifies while still airborne, forming cinder that piles up around the vent to form the cinder cone.
At right, just over the shoulder of the person in the parka, is Horseshoe Crater in the distance. This is another Capulin Phase volcano, relatively young at 238,000 years of age.
Matthew Zimmerer, one of the conference organizers, briefs us on the geology of the Clayton-Raton volcanic field.
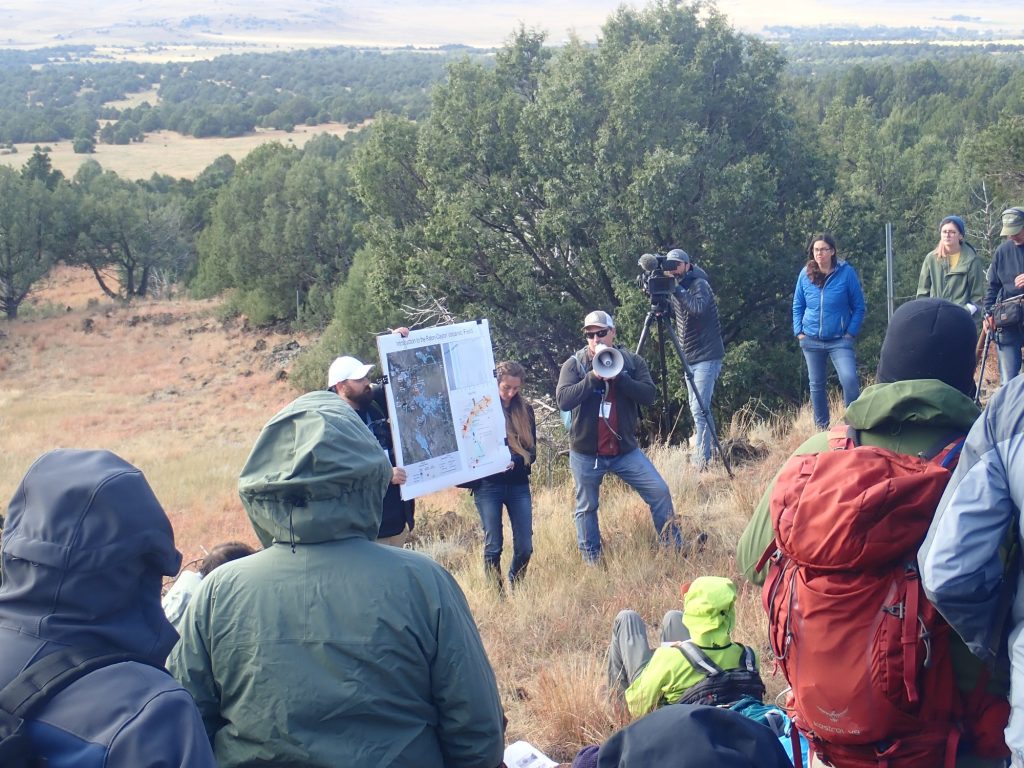
The camera in the background is a team filming for a Park Service movie on Capulin Mountain. While I don’t object to being an extra in a movie, I’m a little too old to get very excited about it, either.
The weather is rather cold and miserable, but will improve considerably by midday.
I’ve been on the lower flow trail before, so I persuade Gary to take the boca trail with me. Eruptions are thought to have begun at Capulin at a small vent on the east side of Capulin Mountain. Activity then shifted to the vent at the center of Capulin Mountain, building the large cinder cone. Subsequent eruptions were effusive (relatively gentle flow of lava out of the ground) and centered on the boca (Spanish “mouth”), a vent at the west base of Capulin Mountain.
When a cinder cone erupts, there is a tendency for fountaining to be followed by effusive eruption of lava as the eruption taps into deeper, less gassy magma. Because the lava (lava is degassed magma that has reached the surface) is more dense than the porous cinder of the cinder cone, the lava often “rafts” part or all of the cinder cone wall away as it flows through the base of the cone. Capulin Mountain is so well preserved, in part, because the effusive phase found a vent at the boca that prevented the main cone from being destroyed by rafting.
On the way back from Sierra Grande Viewpoint, we pass a levee.
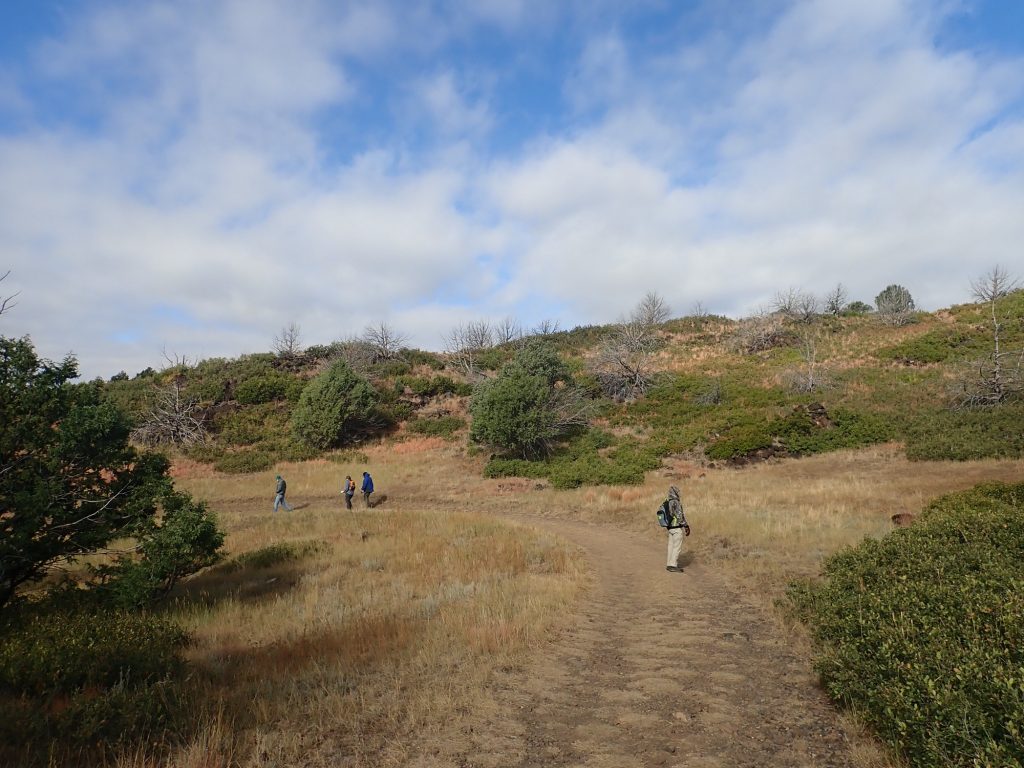
The levee is the small ridge in front of us. It was formed along the margins of a large flow from the boca, where the lava cooled enough to solidify and form a feature very much like a natural levee of a river.
We cross the park road at the barricade (placed because of the ruined road) and start up the boca trail. Looking back at Horseshoe Crater:
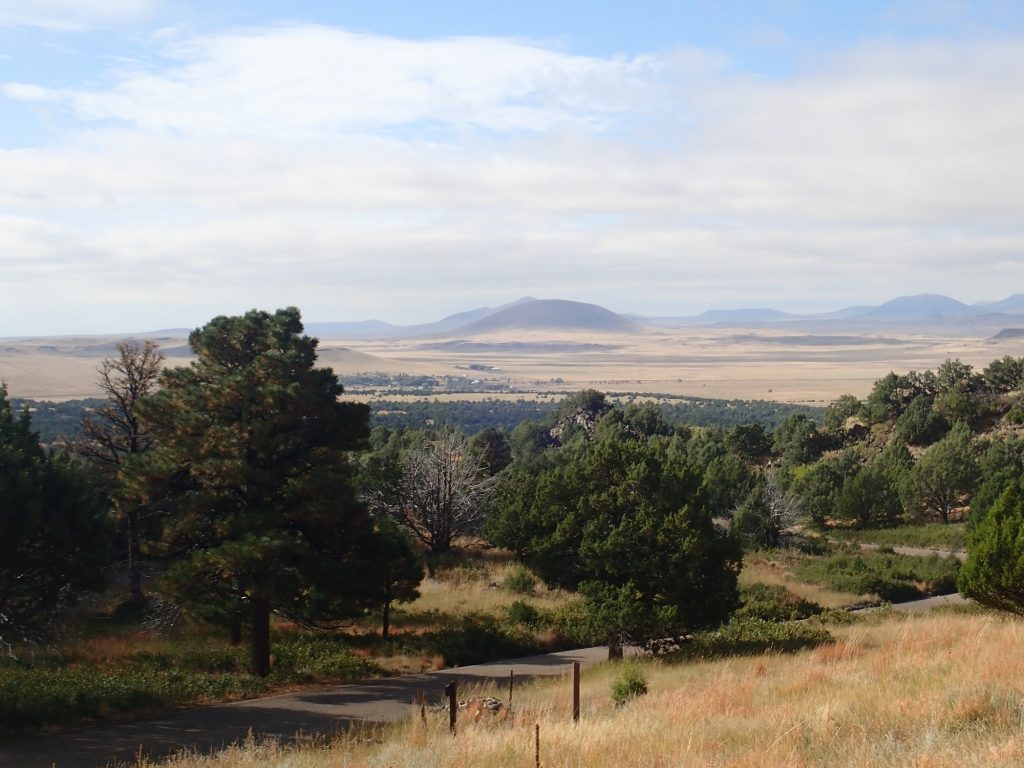
Right of Horseshoe Crater on the skyline is Hogeye Mesa, which is capped with a Clayton phase flow. To its right is Pine Butte East, a Raton phase dome of Red Mountain Dacite.
The trail comes to a small valley.
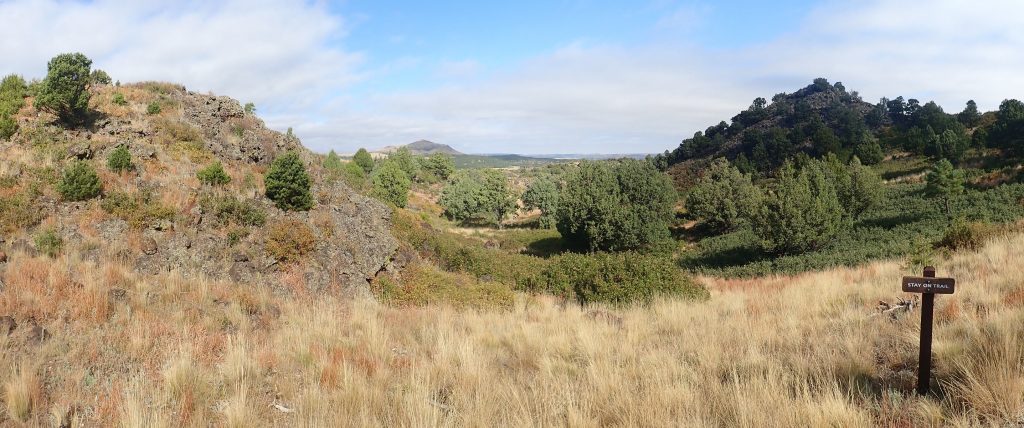
The Park Service map identifies this as one of the two main boca vents. The very rugged hills on either side are spatter, produced by lava thrown into the air that was not completely solidified by the time it fell back to the ground. This produces deposits somewhere between loose cinder and solid rock.
A view from a point on the trail a little higher above the boca.
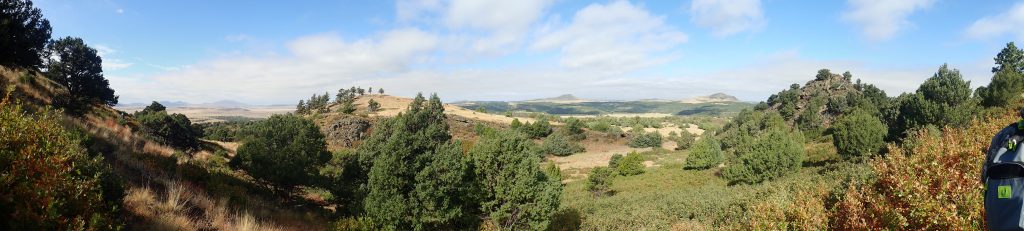
The peaks on the skyline are Jose Butte at left and Robinson Peak at right. Both are Clayton phase volcanoes, and both are composed of highly alkaline volcanic rock. Volcanic rock with this high a ratio of alkali to silica is classified as feldspathoidal, because it does not have enough silica to combine with the alkali to form feldspar, the most common alkali mineral. Instead, the alkali forms feldspathoids, which are structurally similar to feldspar but contain less silica in their formula. For example, albite (sodium feldspar) has the formula NaAlSi3O8, while nepheline (sodium feldspathoid) has the formulat NaAlSiO4. The Raton-Clayton field is notable for the frequent occurrence of highly alkaline volcanic rock.
Next is a collapsed lava tube leading north from the second boca vent.
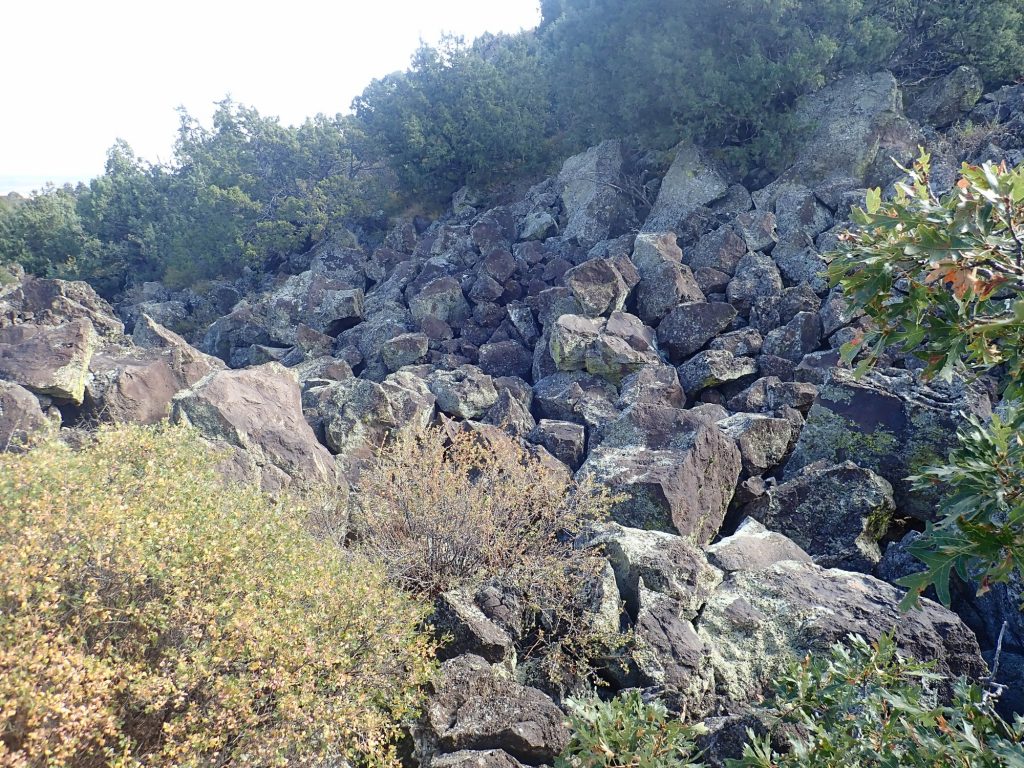
A lava tube forms when the sides and surface of a lava flow are able to cool enough to solidify. Molten lava continues to flow through the tube thus formed, and the solid lava of the walls and roof act as pretty good insulation, allowing liquid lava to flow further from the cone than it could as an exposed flow. When the eruption ends, the tube drains, and the loss of support for the roof often causes it to collapse. This has taken place here, and the basalt boulders are the remnants of the tube roof. You can see the layers of the roof on the other side of hte collapsed tube.
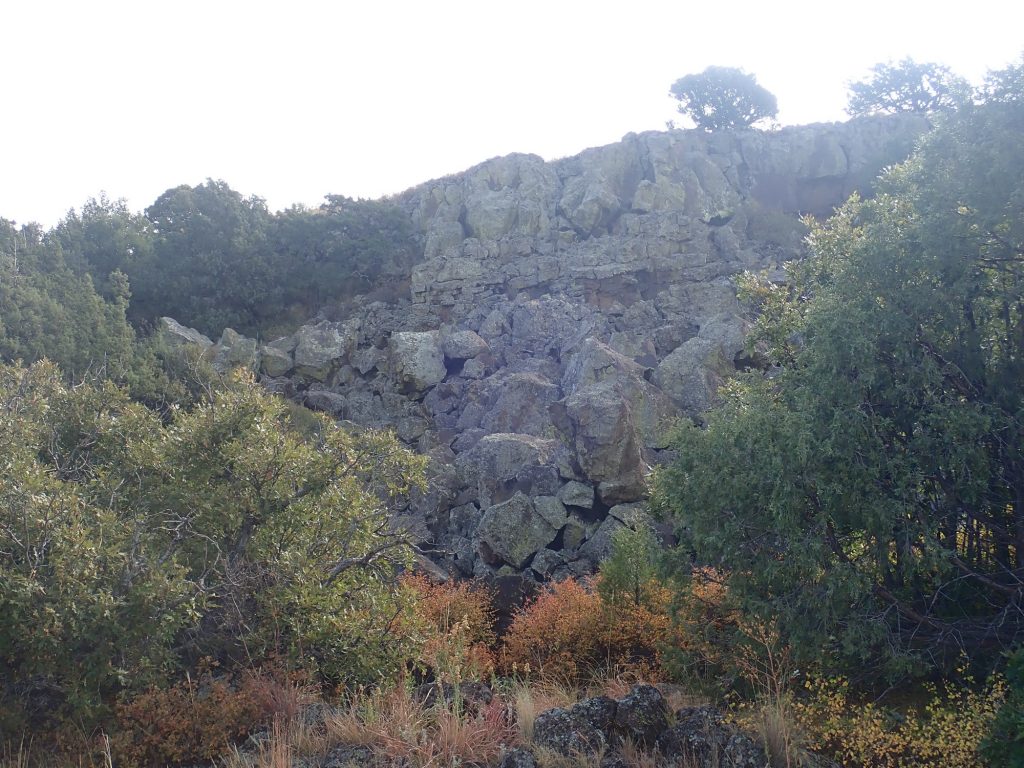
This tube was an important conduit for lava from the second boca vent, which is to our right here.
The trail turns west and reaches what looks like another collapsed lava tube or old lava flow with levees. In the distance is Mount Robinson.
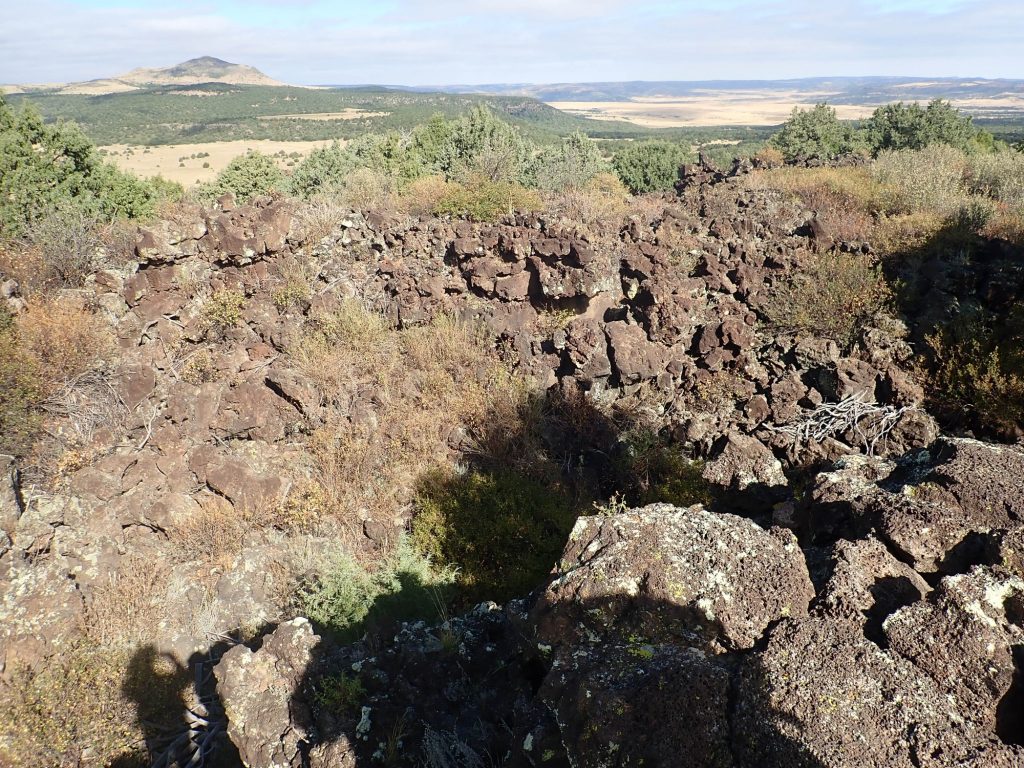
Here the trail crosses part of a lava lake that formed near the boca.
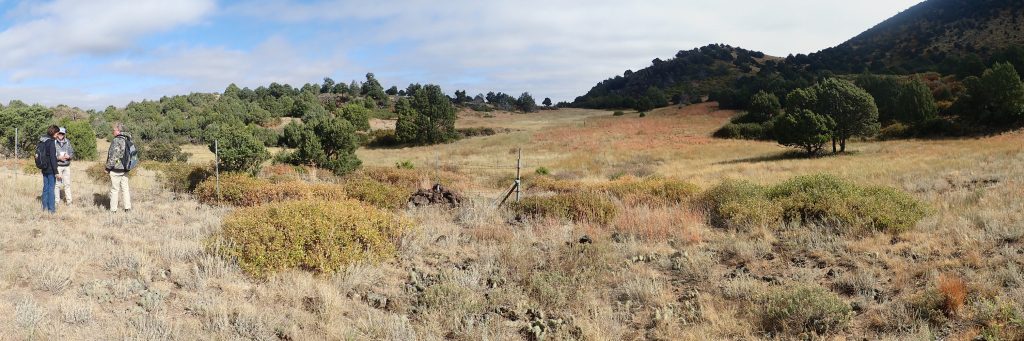
Next is Aspen Grove Overlook.
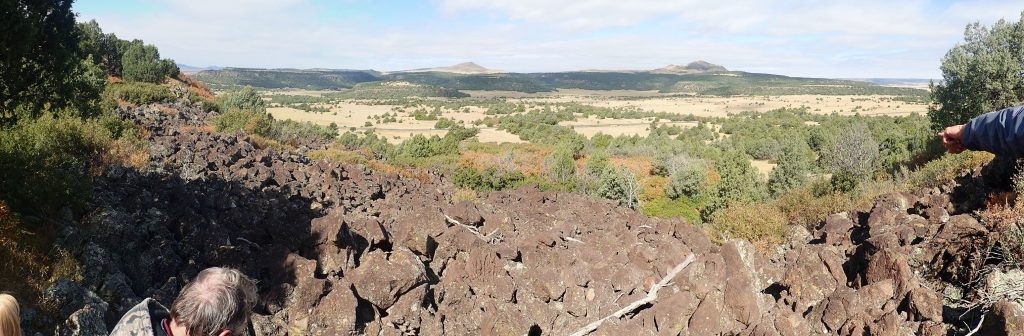
Not that much of a grove, but you get a nice view of Jose Butte and Robinson Peak sitting on a thick flow of Clayon phase basalt or basanite.
Basalt is an extremely common low-silica volcanic rock composed of plagioclase feldspar, pyroxene, and accessory minerals. Basanite differs in having a higher akali content, so that much of the feldspar is replaced by feldspathoids, and enough magnesium is present for olivine to be part of its normative composition. Basanite is much less common than basalt, but is found in several locations in the Clayton-Raton field.
A volcanic chimney.
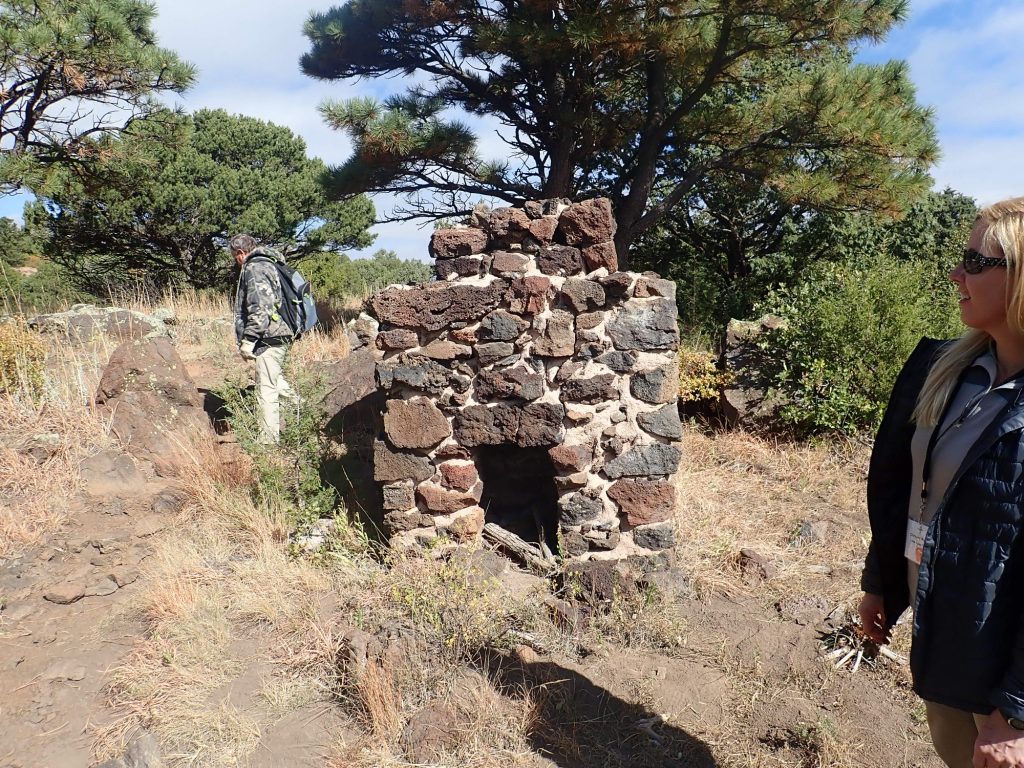
🙂 Apparently emplaced by the Civilian Conservation Corps in the early days of Capulin Volcano National Monument.
Then back to the visitors’ center parking lot, where it turns out our lunch has not arrived. Apparently it got sent to the wrong location. With apologies to Yakov Smirnov: “In northeastern New Mexico, lunch loses you.”
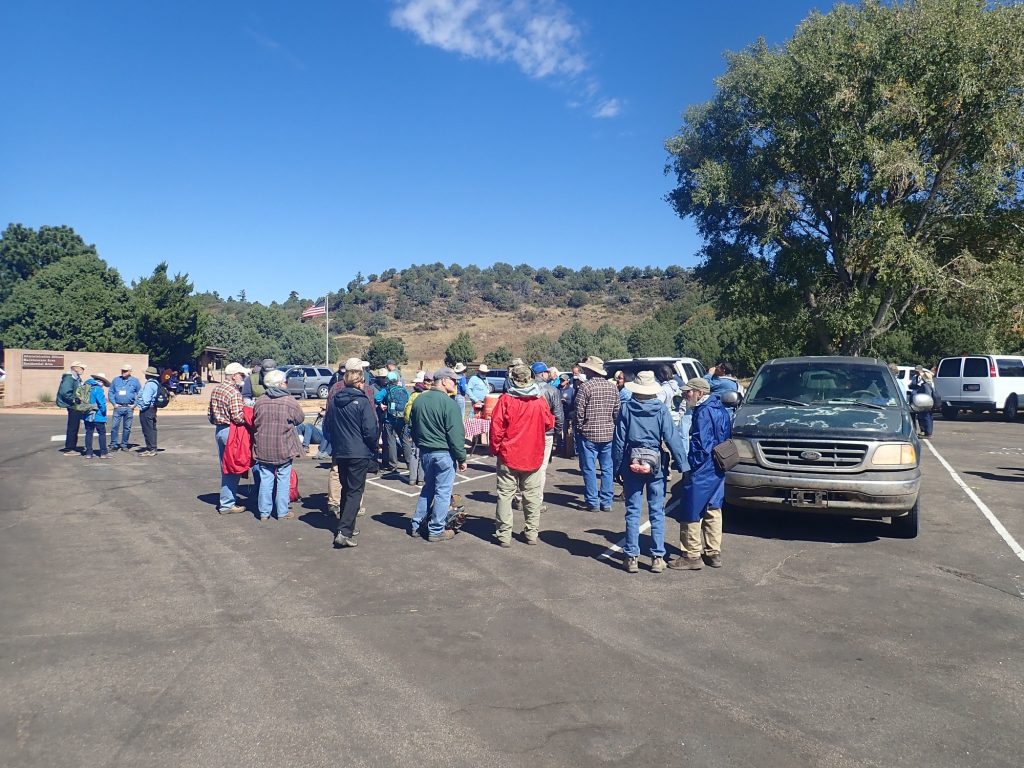
We drive the short distance north to Mud Hill.
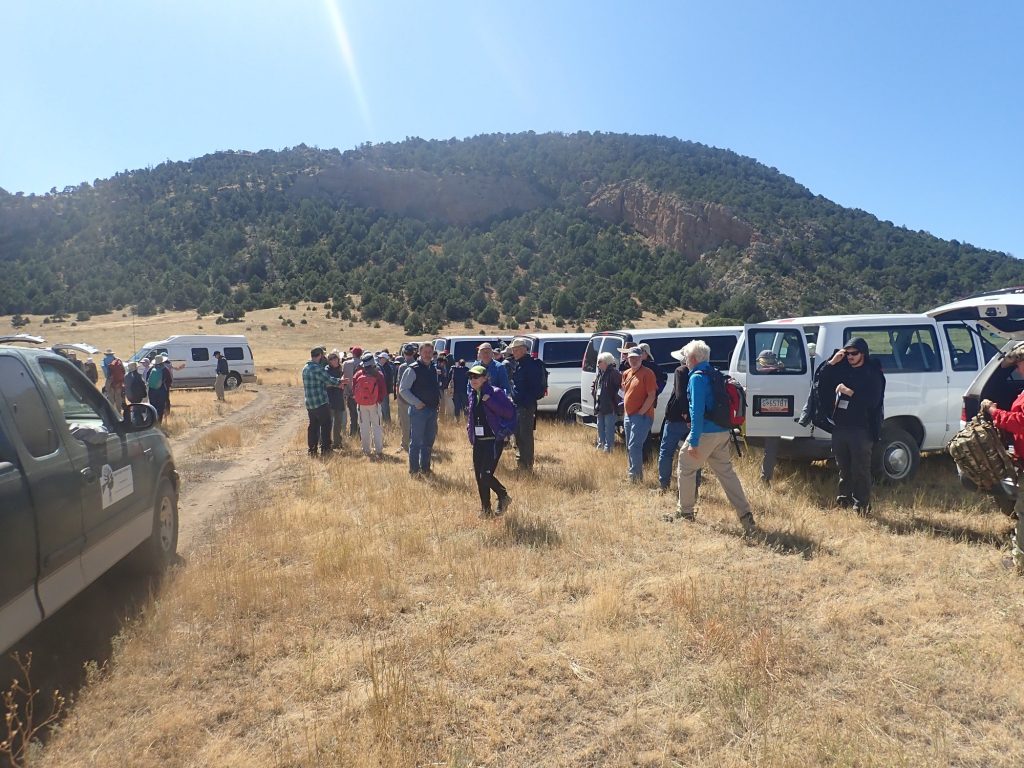
It really does look like mud.
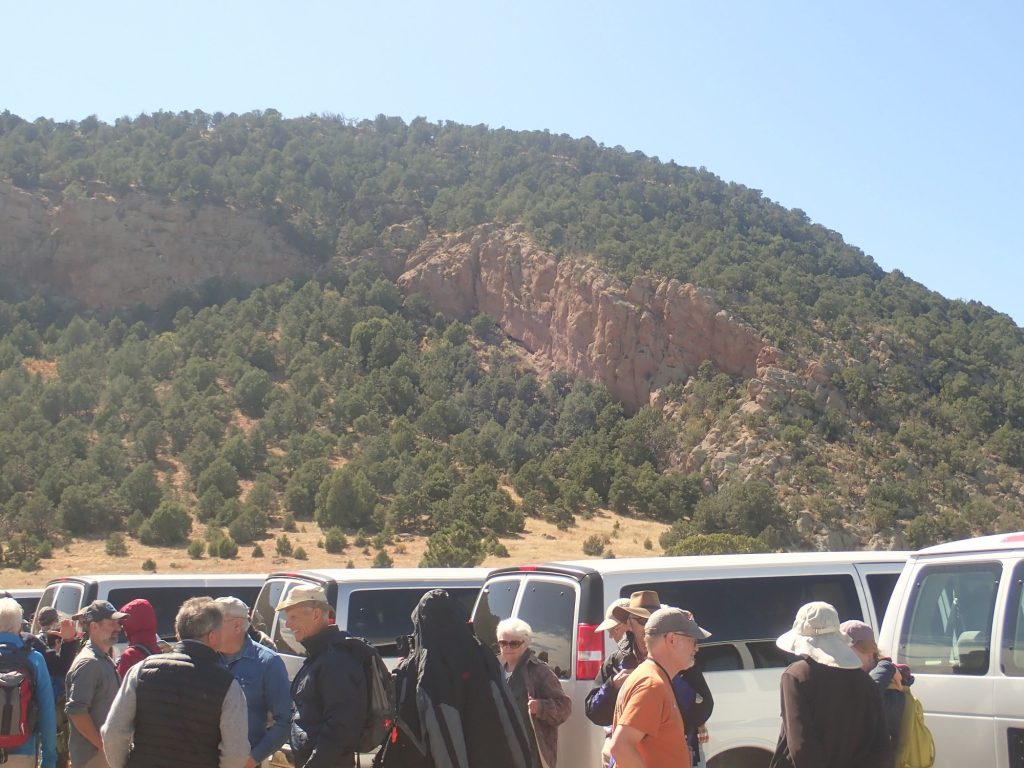
But, as we will shortly discover, it’s quite hard rock. We eat lunch
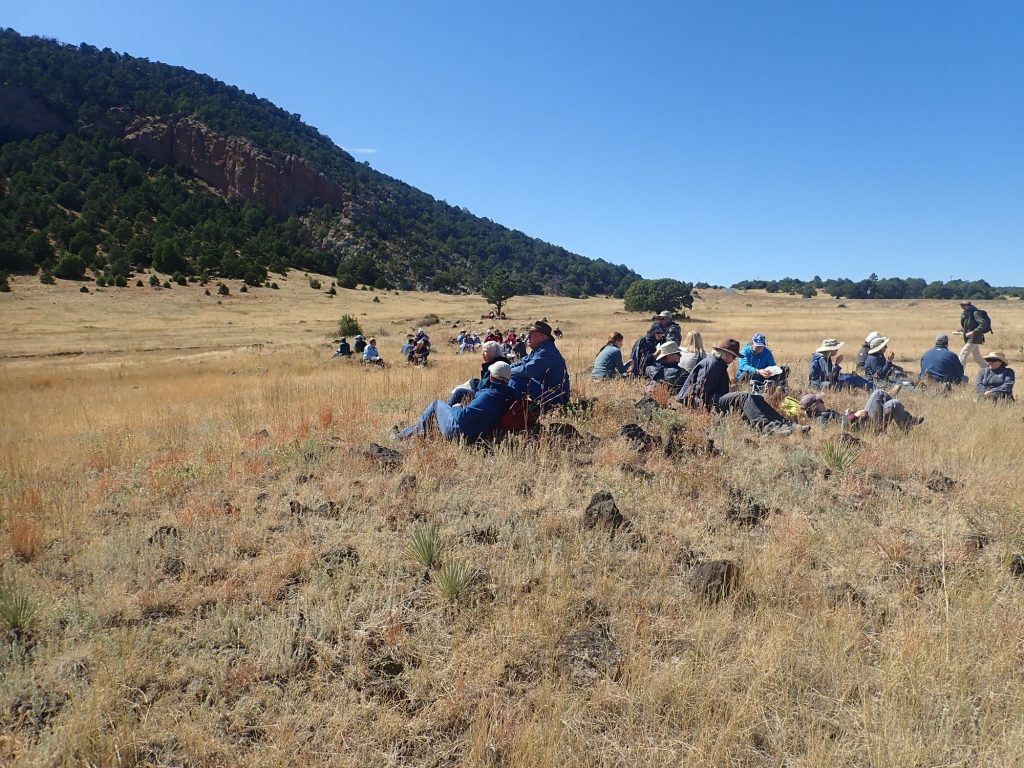
then troop off to inspect this feature.
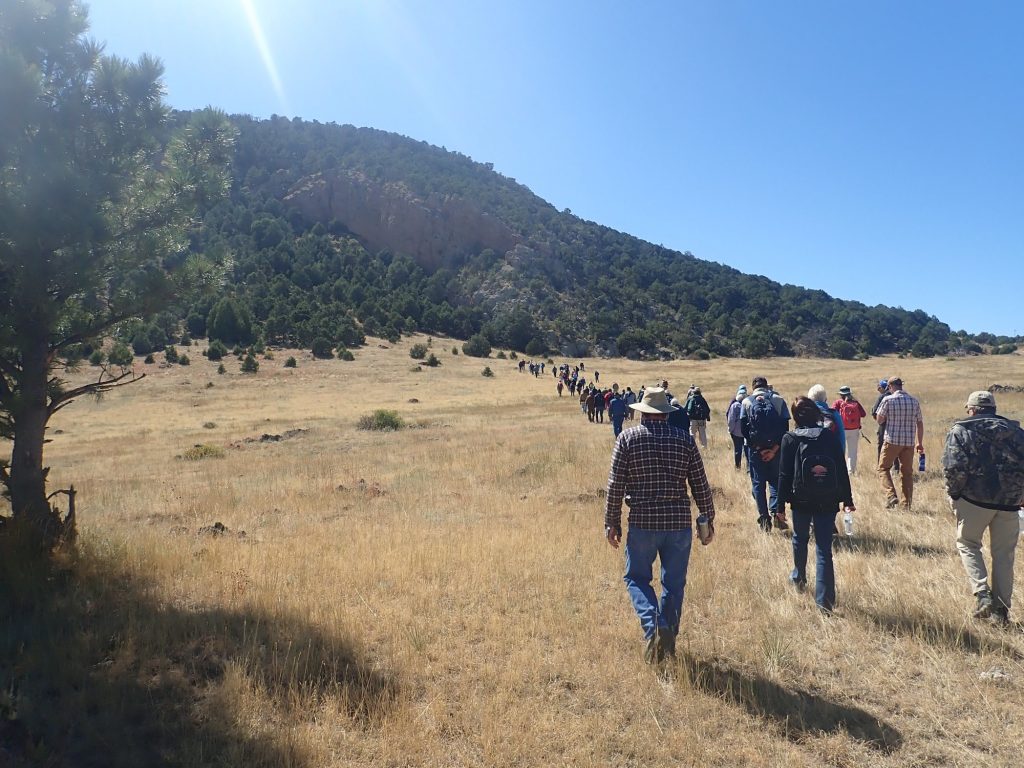
Matthew Zimmerer again briefs us.
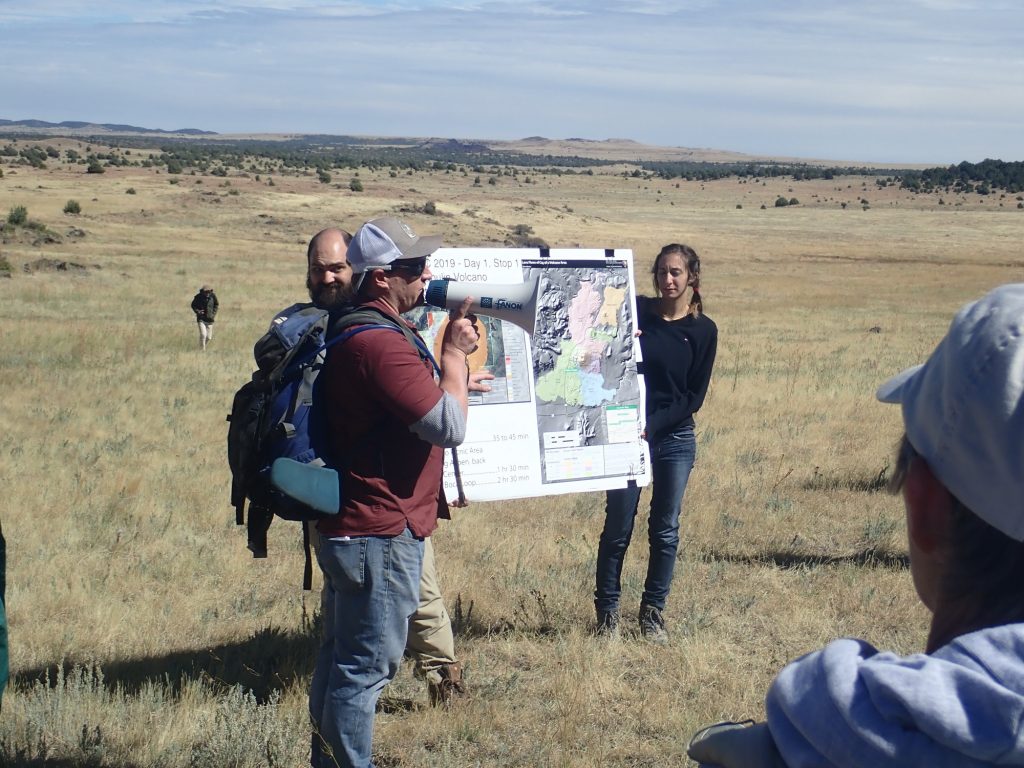
Mud Hill is considerably older than either Capulin to the south or Baby Capulin to the north at about 1.7 million years in age. This would be very earliest Capulin phase of the volcanic field. It is a tuff cone, formed when magma erupts through wet sedimentary beds or shallow water (a phreatomagmatic eruption.) It takes just the right amount of water: Any more, and the eruption would have produced pillow basalt; any less, and the eruption would have produced a cinder cone like Capulin.
Nearby is a freshly broken clast showing what the phreatomagmatic rock is like.
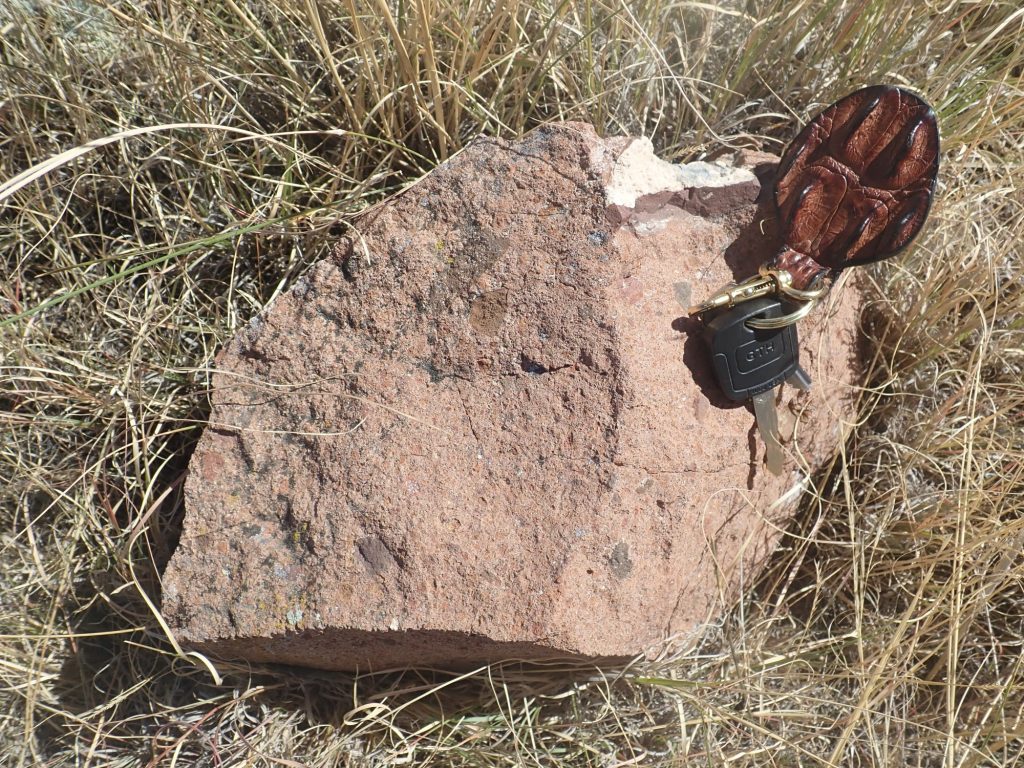
You can see a few dark clasts of country rock, the rock through which the volcano erupted, and considerable pinkish fine-grained matrix. This formed from magma and country rock that was pulverized by the steam explosions accompanying the eruption.
Another clast shows cemented lapilli.
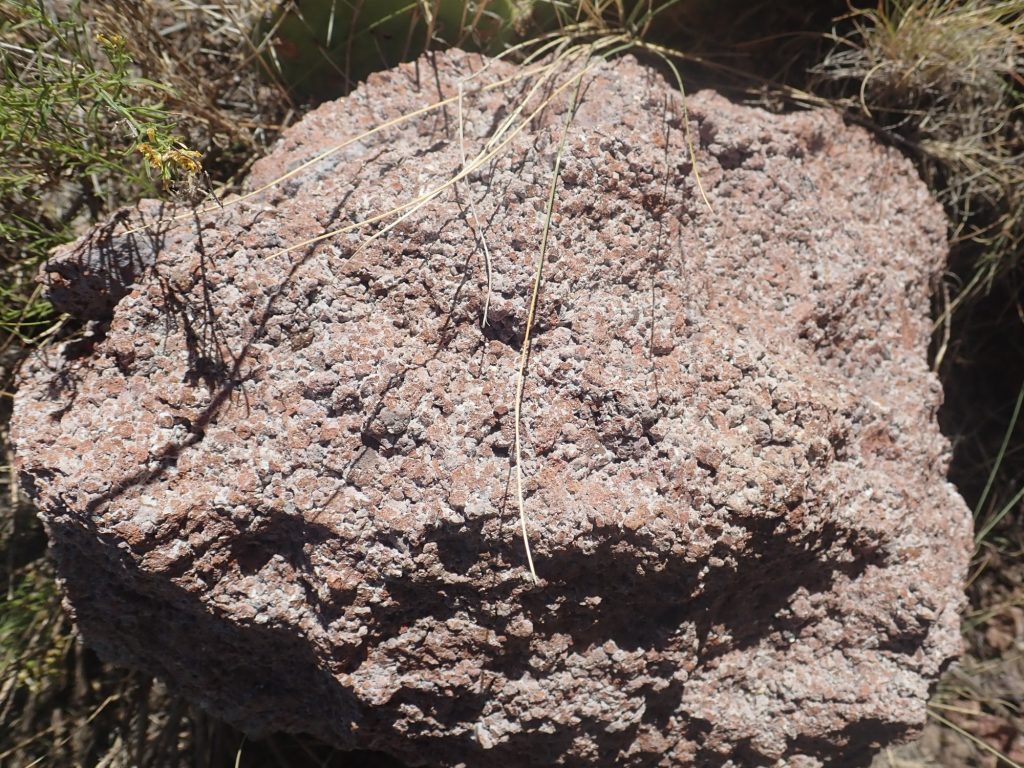
Lapilli is intermediate-sized tephra, broken rock thrown out of a volcanic vent. The finest tephra, under 2mm in size, is called ash, while tephra over 64mm in size is called volcanic blocks or volcanic bombs depending on whether the rock was completely solid when it came back to earth. (Bombs are not completely solidified.) Matthew Zimmerer invited us to try to determine whether the lapilli here was accretionary or not. Accretionary lapilli is formed from fine ash that aggregates into larger blobs. I think it occurred to many of us that an accretionary lapillus would show an onion ring structure if cut in half, but none of us had the equipment to do this.
Exploring the outcrops, which appear to have numerous bits of calcite or zeolite filling bubbles in the original rock.
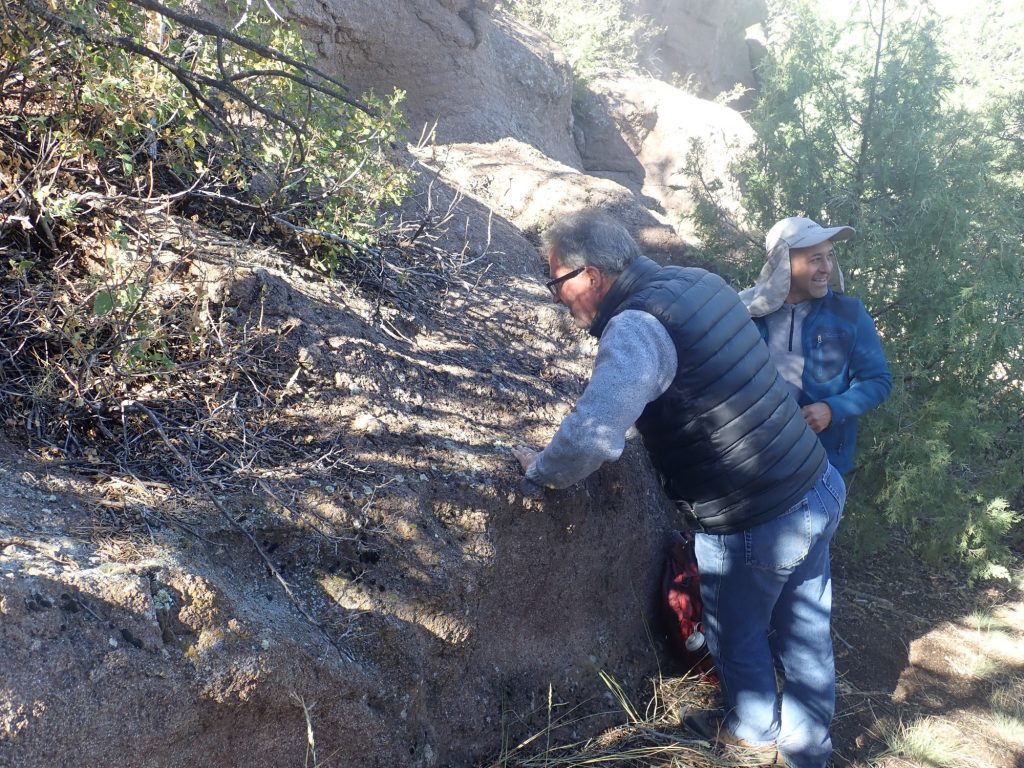
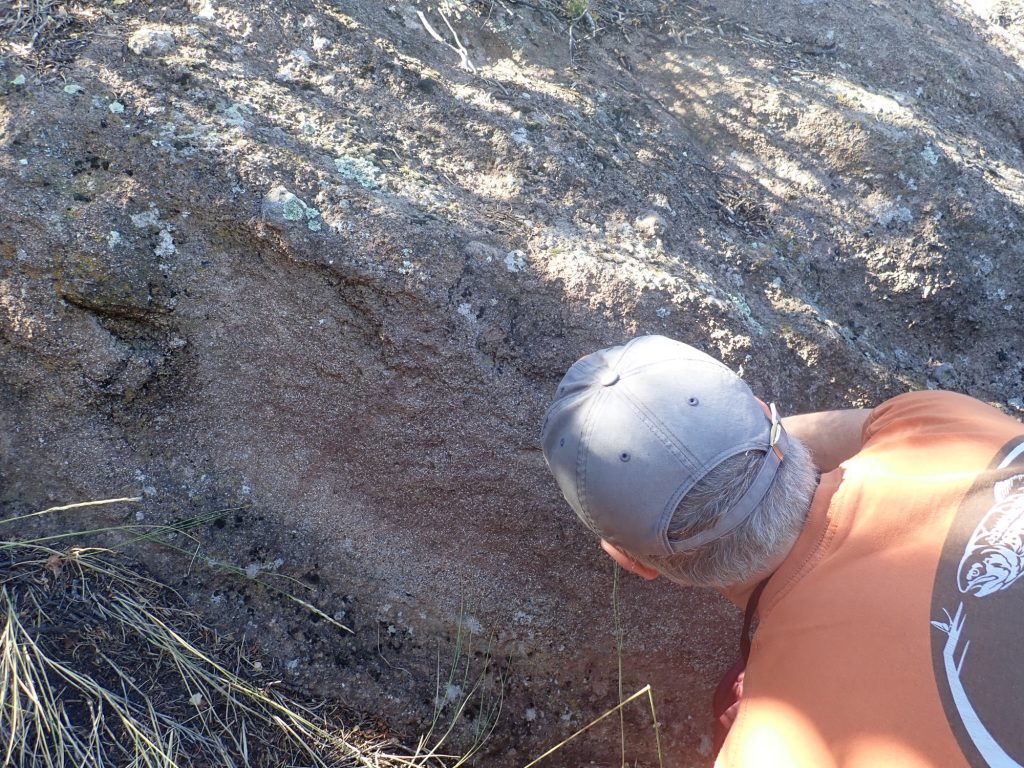
Here an outcrop catches the light just right to emphasize its bedding. Too bad the sun is so badly backlighting the lens of my camera.
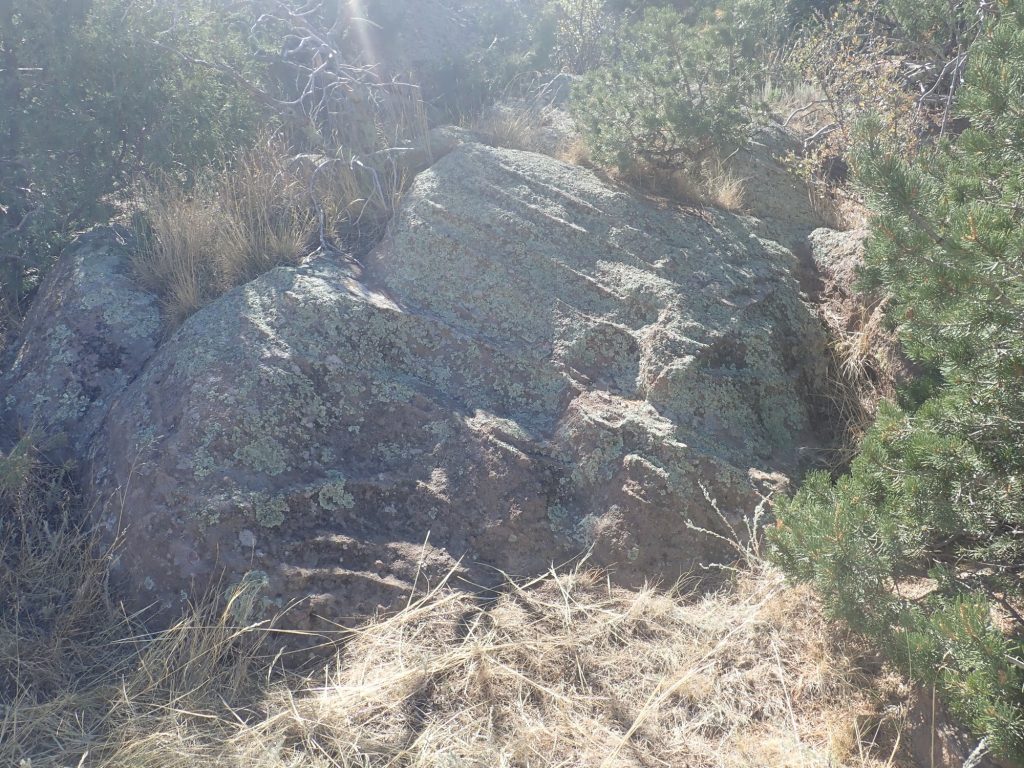
An arroyo exposes more phreatomagmatic beds.
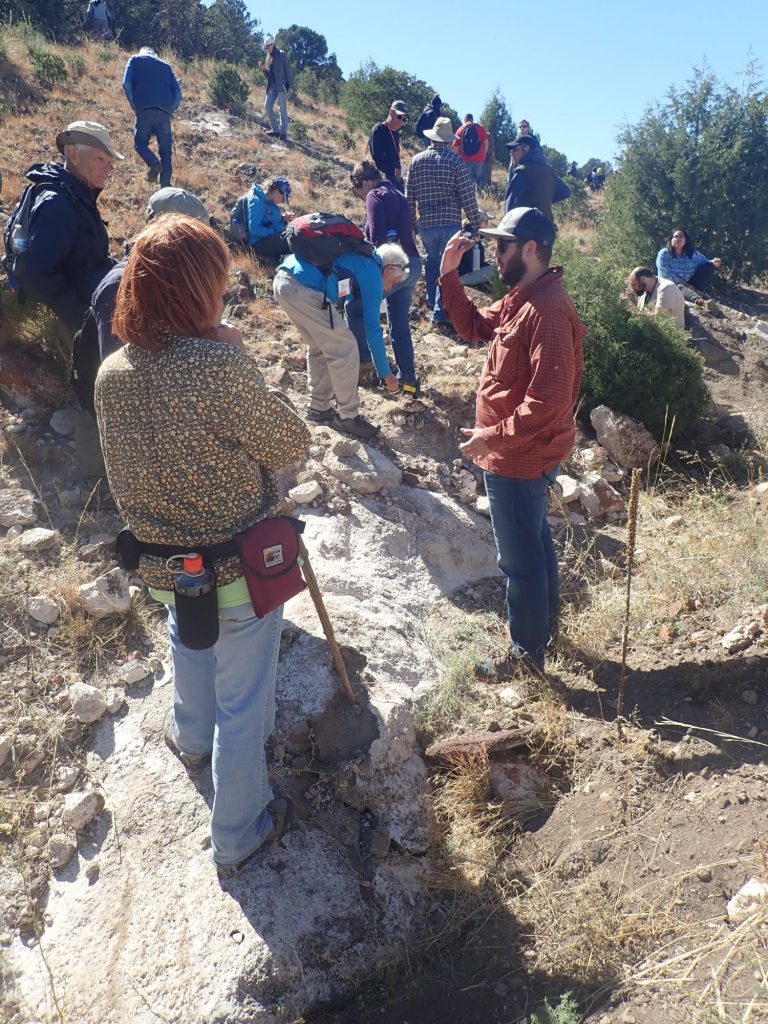
I persuade Gary to pose with a volcano.
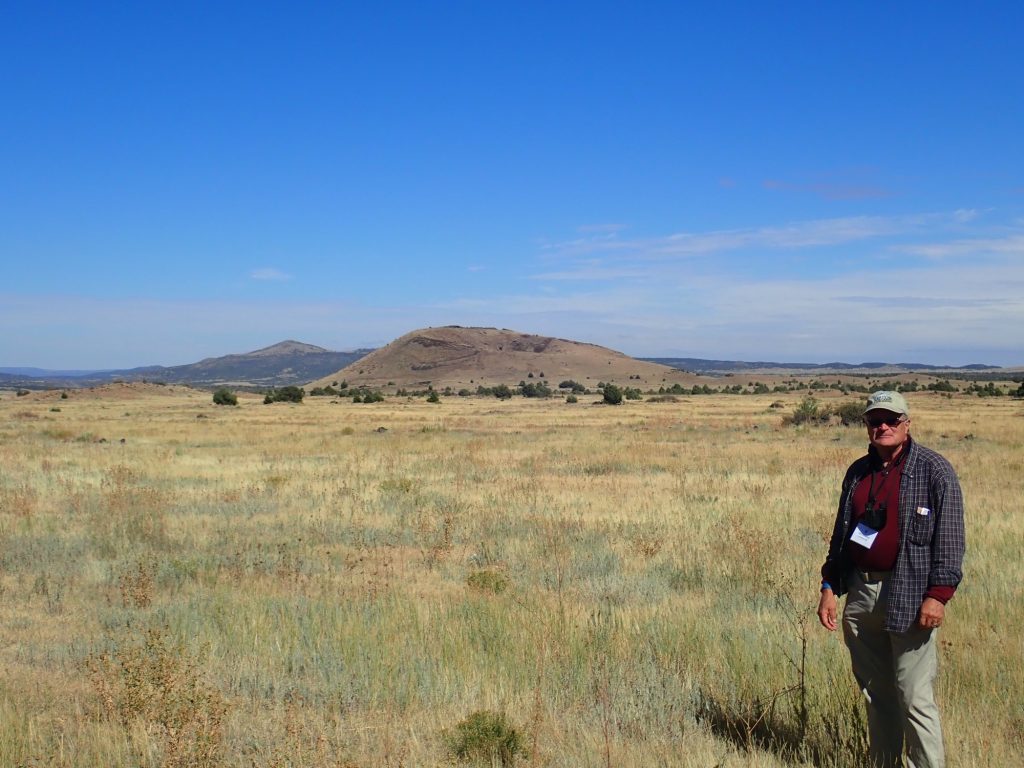
This is Baby Capulin, which is about 37,000 years old — among the youngest in the Raton-Capulin field. It is our next stop.
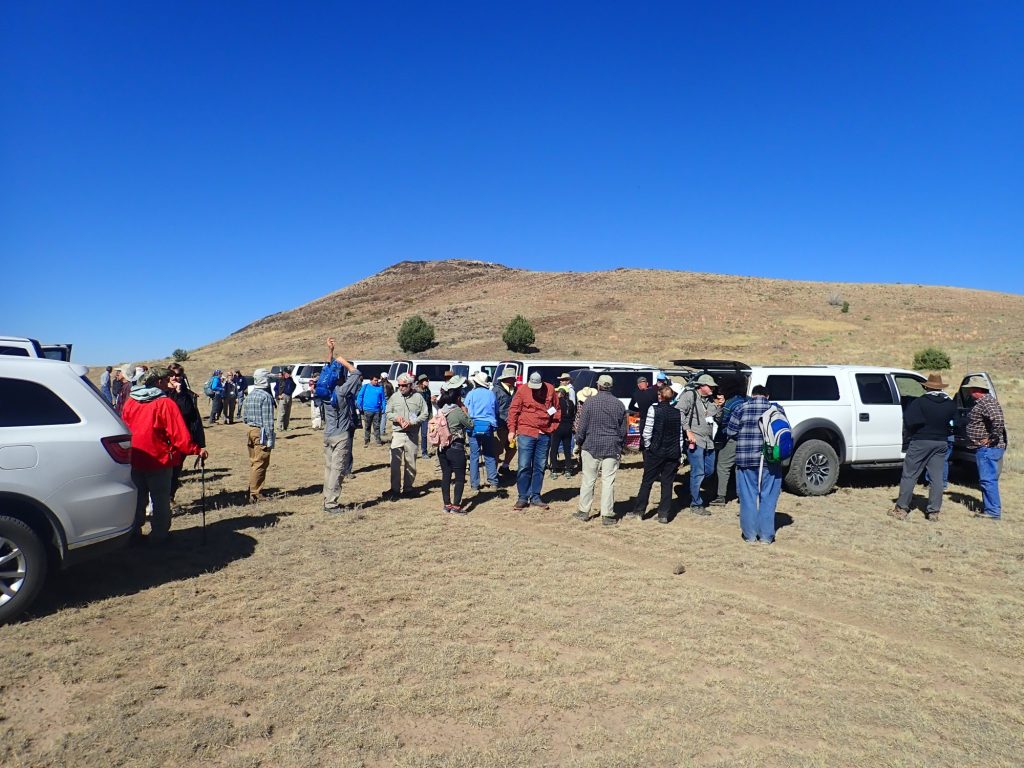
Another presentation, this time by Matthew Zimmerer and Frank Ramos.
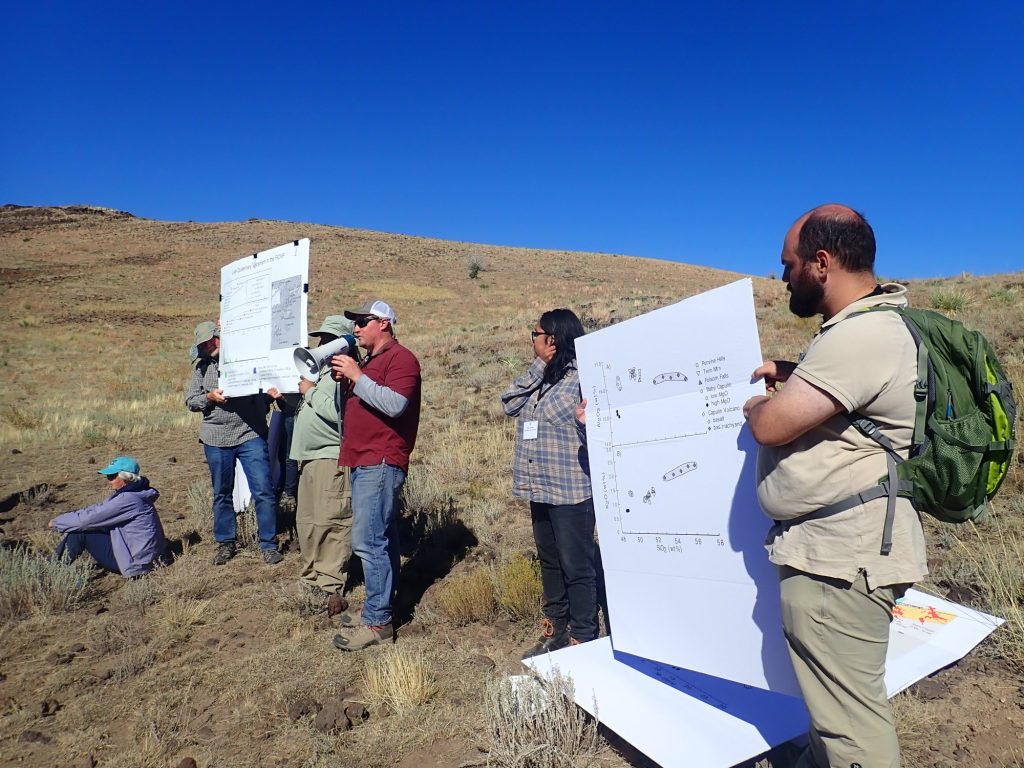
Most of the lava from the Capulin phase follows the same trend lines in the major element versus silica diagrams. However, one of the flows from Baby Capulin is distinctly more primitive than the others, and may be the best indicator of the nature of the mantle under this area.
The Earth’s crust, its outermost layer, forms a thin skin, accounting for less than 1% of the Earth’s volume. Like human skin, which varies in thickness from the very thin skin of the eyelids to the thick skin of the soles of the feet, the Earth’s crust varies in thickness from about 3 miles thick in some ocean basins to 60 miles thick in mountainous regions.
The crust is composed of relatively low-density, high-silica minerals, of which feldspar is the most characteristic. Quartz, a pure silica mineral, is also important. This light material floats on the mantle, which makes up the bulk of the Earth’s volume and is composed of more dense, silica-poor, magnesium-rich minerals, of which olivine is the most characteristic.
We can locate the crust-mantle boundary from the behavior of seismic waves, which abruptly speed up when they penetrate the mantle. The boundary between the two is known as the Mohorovičić discontinuity, after the Croation seismologist who first recognized it. Most English-speaking geologists call it simply the Moho or the crust-mantle boundary.
The upper mantle is not entirely uniform. The uppermost part is rigid rock, like the crust. The crust and rigid outermost mantle are known as the lithosphere. Further down, as the temperature rises, the mantle melts slightly — not more than a few percent of its volume. However, this is enough to make the rock at this depth much weaker, giving it its name — the asthenosphere, from the Greek asthenḗs = ‘weak’. Continental drift is possible because, over very long time scales, the aesthenosphere acts as a ductile layer that allows slippage between lithosphere and the deeper mantle.
The slightly melted aesthenosphere seems like a likely candidate for the origin of magmas, and geologist believe that silica-poor magmas do indeed originate here. The mantle rock is very low in silica and very high in magnesium, but only a small percentage is ever melted, and the material that melts first is higher in silica, much lower in magnesium, and is highly enriched in the alkali elements, sodium and potassium. This is very nearly the composition of alkaline basalt, and so geologists believe alkaline basalts like those of the Raton-Clayton field were produced by a low degree of partial melting of mantle rock. A higher degree of melting produces less alkaline magma, and the relatively high percentage of mantle melted at mid-ocean ridges produces low-alkali tholeiitic basalt.
Magma freshly melted from mantle rock is known as primitive magma. Because it is lighter than the rock from which it melted, it is buoyant, and it moves towards the surface. Sometimes there is a clear path for it to reach the surface almost unaltered, and we see low-volume eruptions of highly alkaline magma. More often, the magma stagnates while still deep in the crust, collecting into a magma body that is no longer buoyant because it is surrounded by low-density crust rock.
Much of this magma never reaches the surface, solidifying while still underground. But solidification begins with crystallization of low-silica minerals like olivine. These minerals slowly settle out of the remaining liquid magma, leaving it richer in silica and lower in density. The heat of the magma may also melt surrounding high-silica crustal rock that mixes with the primitive magma. The effect is to restore buoyancy to the magma, which resumes its motion towards the surface as a more evolved magma. Highly evolved magma erupts as andesite, which has about 10% more silica by weight than basalt. Truly high-silica magmas, the kind that produce dacite or rhyolite, are probably the result of extensive melting of crustal rock.
Most of the eruptions of the Capulin phase were of magma that was slightly evolved. The one flow from Baby Capulin may represent nearly primitive magma from which geologists hope to get clues to the nature of the mantle underlying the Great Plains.
Naturally, we all have to take a look at the volcano.
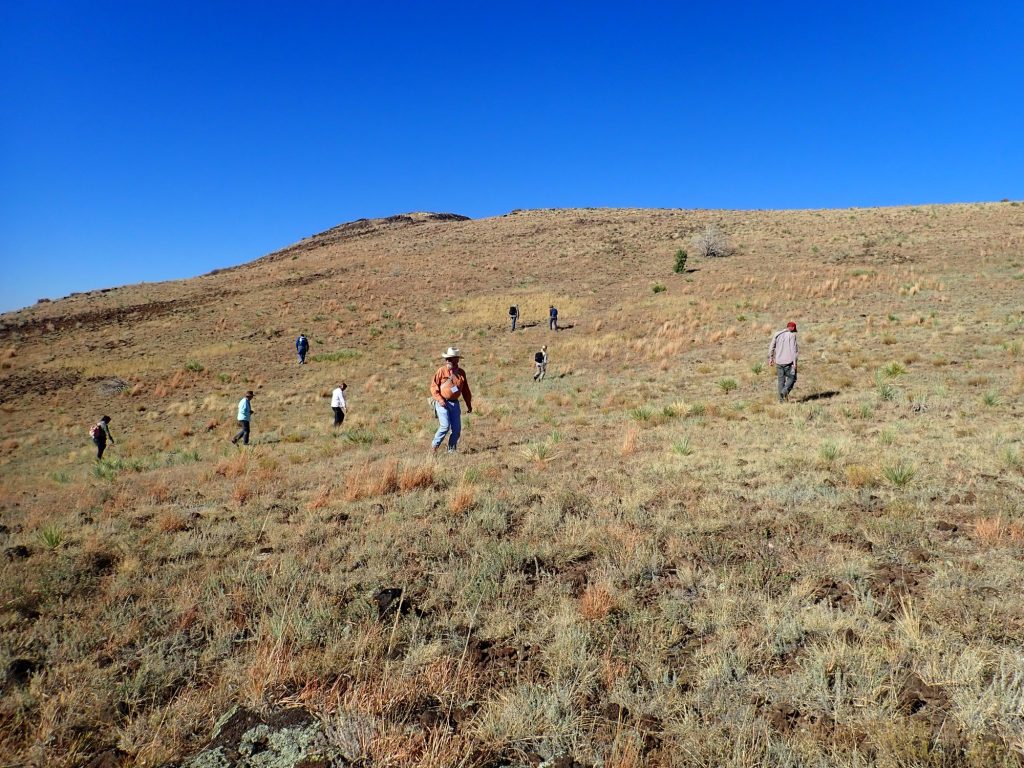
Deep shot of Robinson Peak to the west.
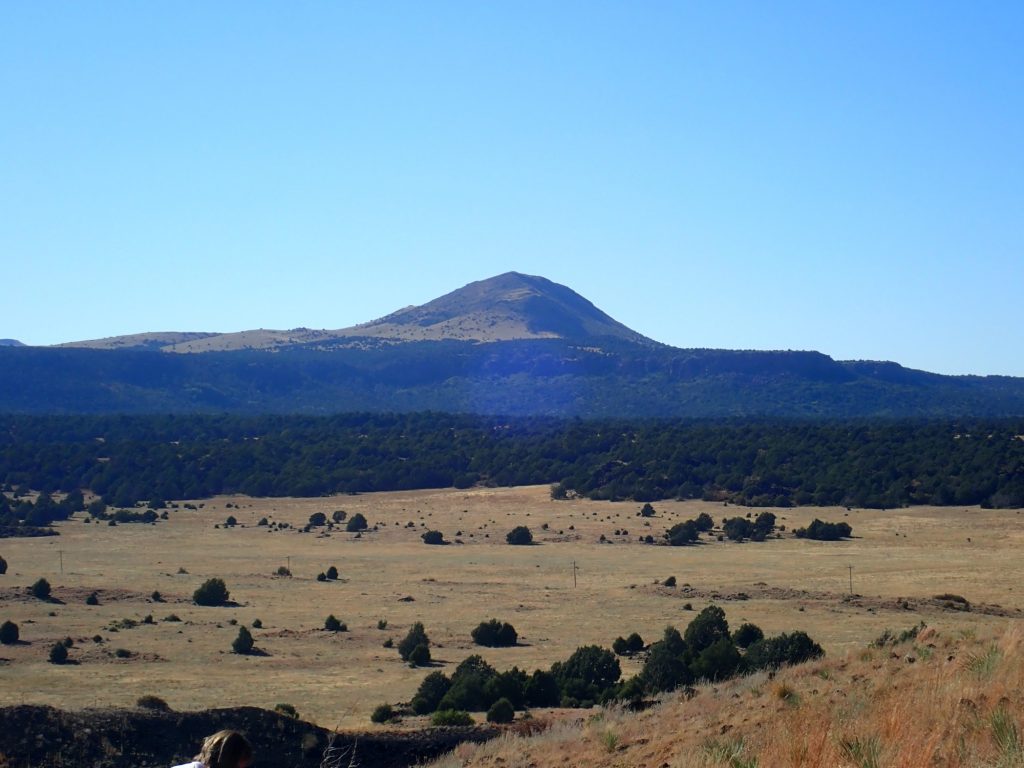
Frank Ramos has challenged us to look for crystals of three characteristic minerals in the rocks we examine. Olivine is a green to brown glassy mineral; pyroxene is a black mineral tending to form oblong crystals that break along flat surfaces; and plagioclase feldspar is a light-colored mineral that also breaks along flat surfaces.
Gary examines a specimen with some help from a geology student.
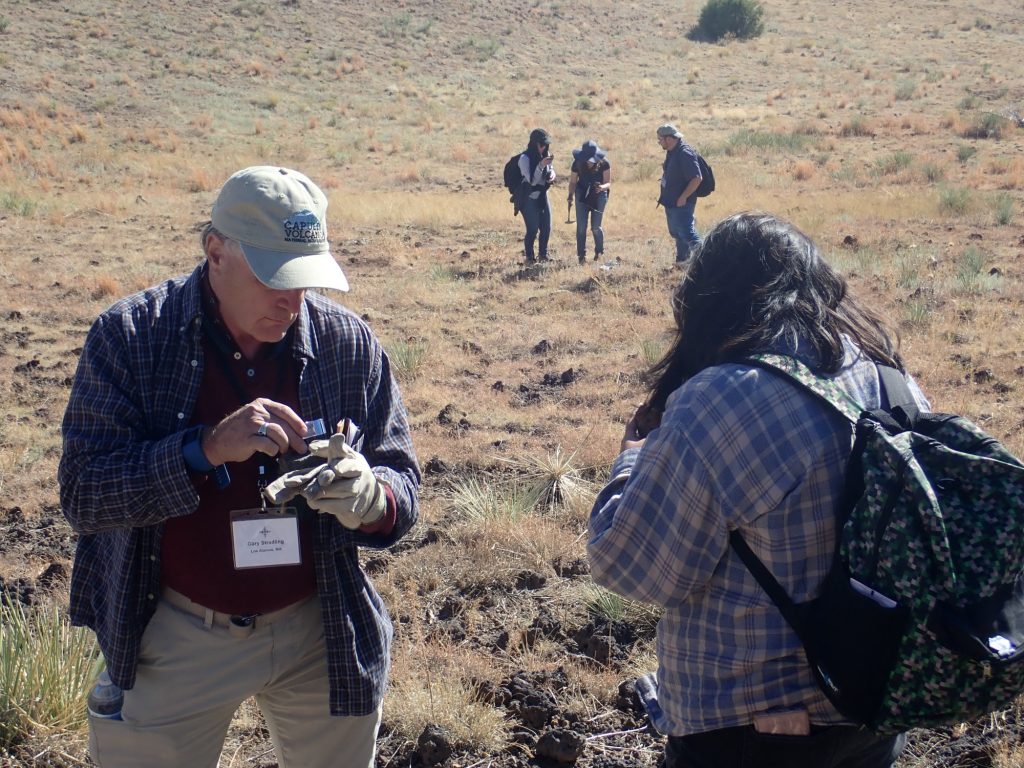
We had no difficulty at all finding olivine; there were a fair number of crystals in the rock we looked at. Pyroxene and plagioclase were more difficult, particularly plagioclase. This is unsurprising in so primitive and alkaline a volcanic rock, which would not be expected to have much plagioclase.
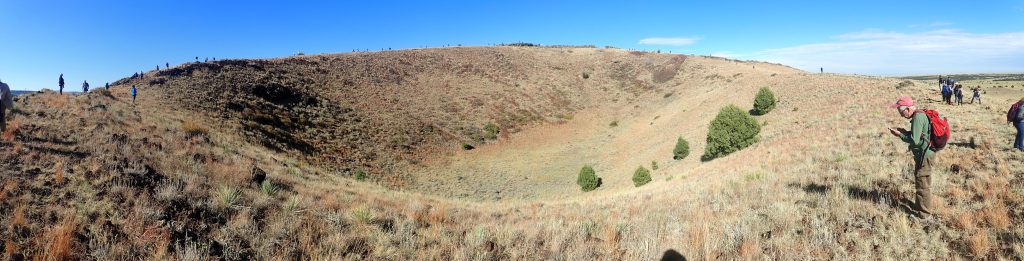
I work my way around the rim, to where we are told some of the youngest beds may be found. Most of the rim is a mix of cinder and splatter.
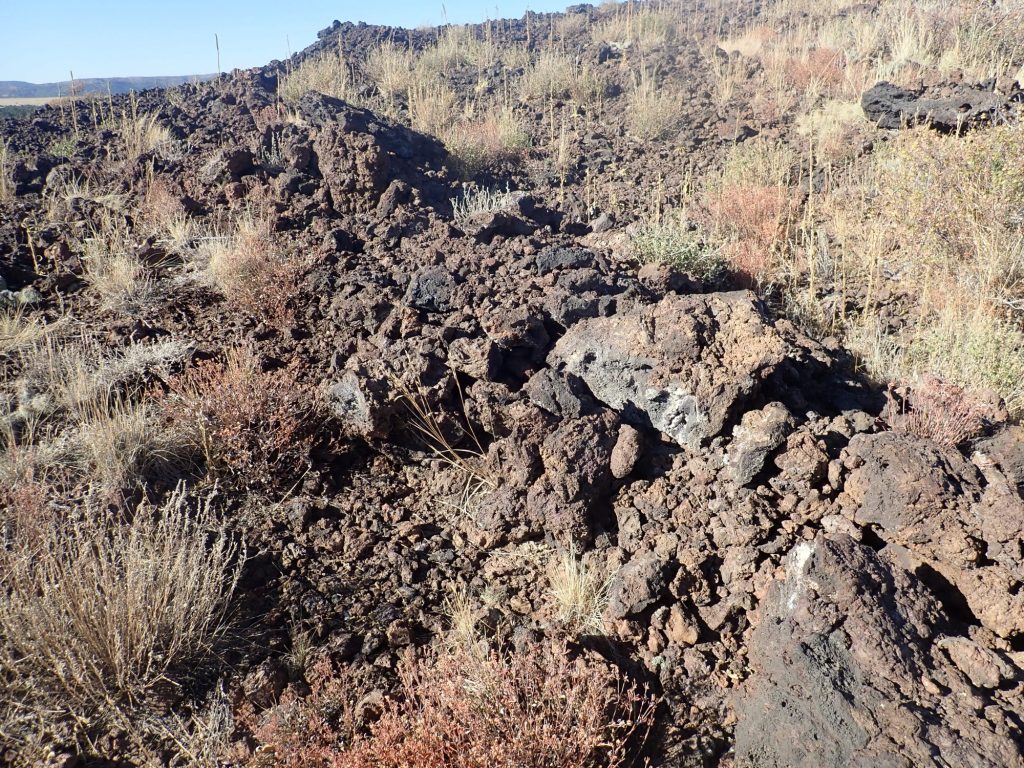
I couldn’t decide if this was a natural feature or a small borrow pit. There are indications of an old jeep road around the rim.
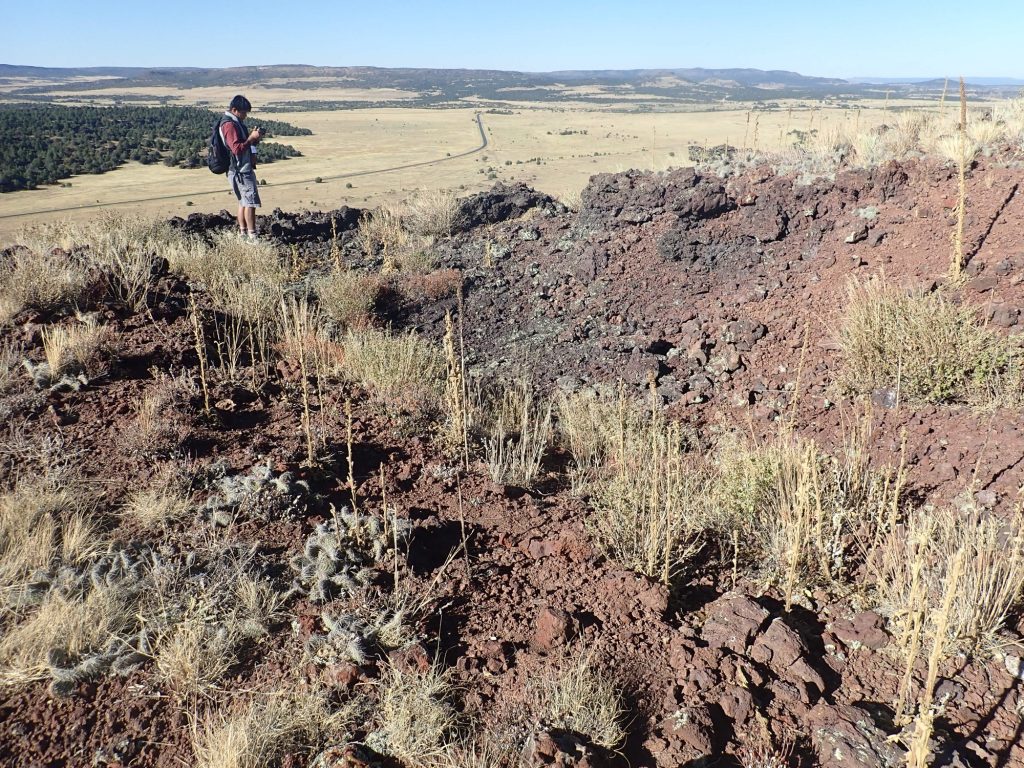
On the other hand, the road does not seem to reach this part of the rim, and this looks like a slump on satellite.
The north rim.
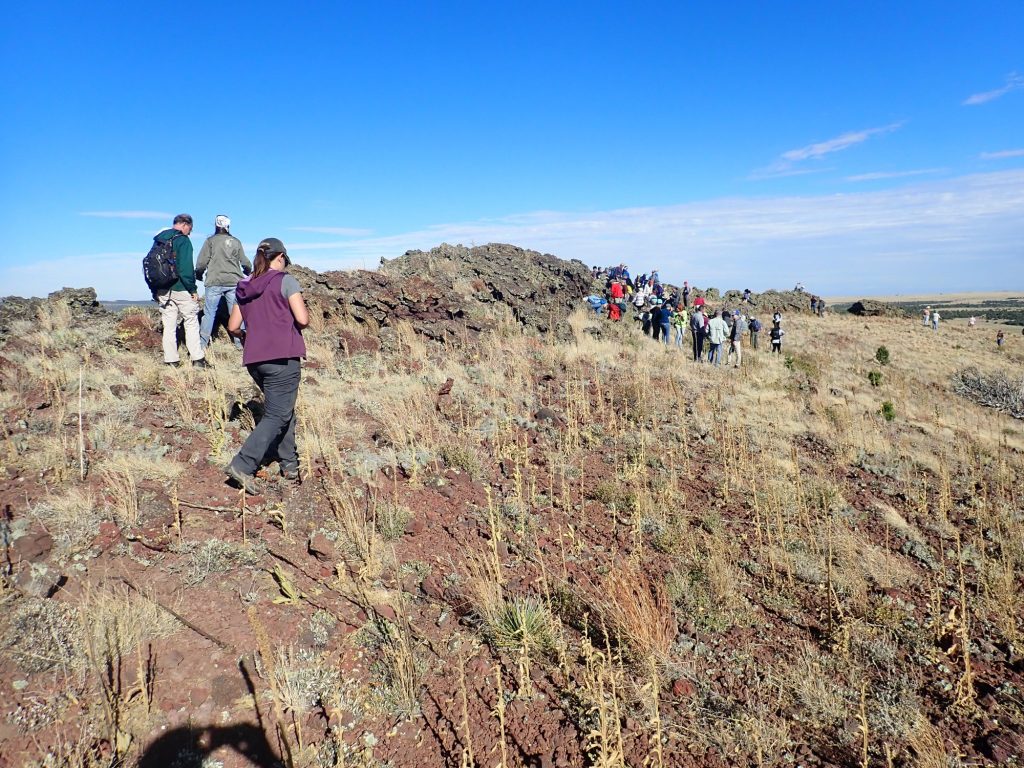
The view to the north.
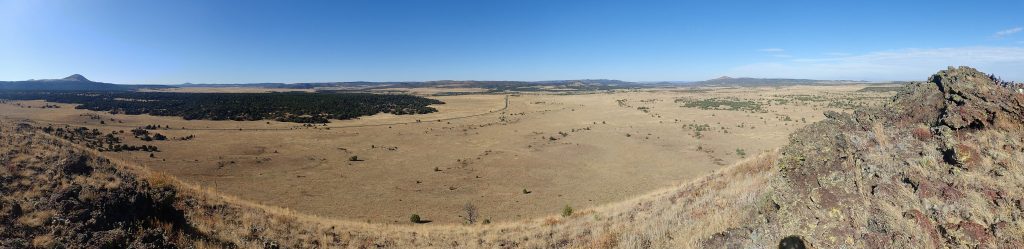
Much of the rim splatter here is glassy.
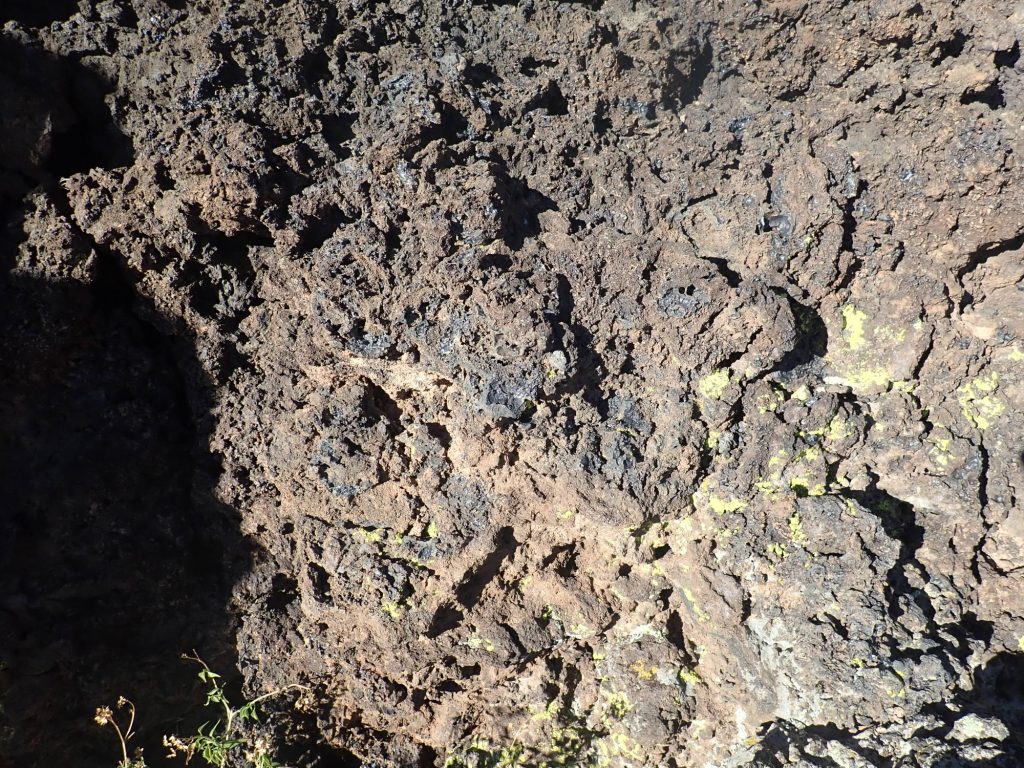
The lava here cooled quickly enough that the tangle of silica in the liquid rock was unable to sort itself out into crystals before solidifying.
We return to the vans and drive to Johnson Mesa.
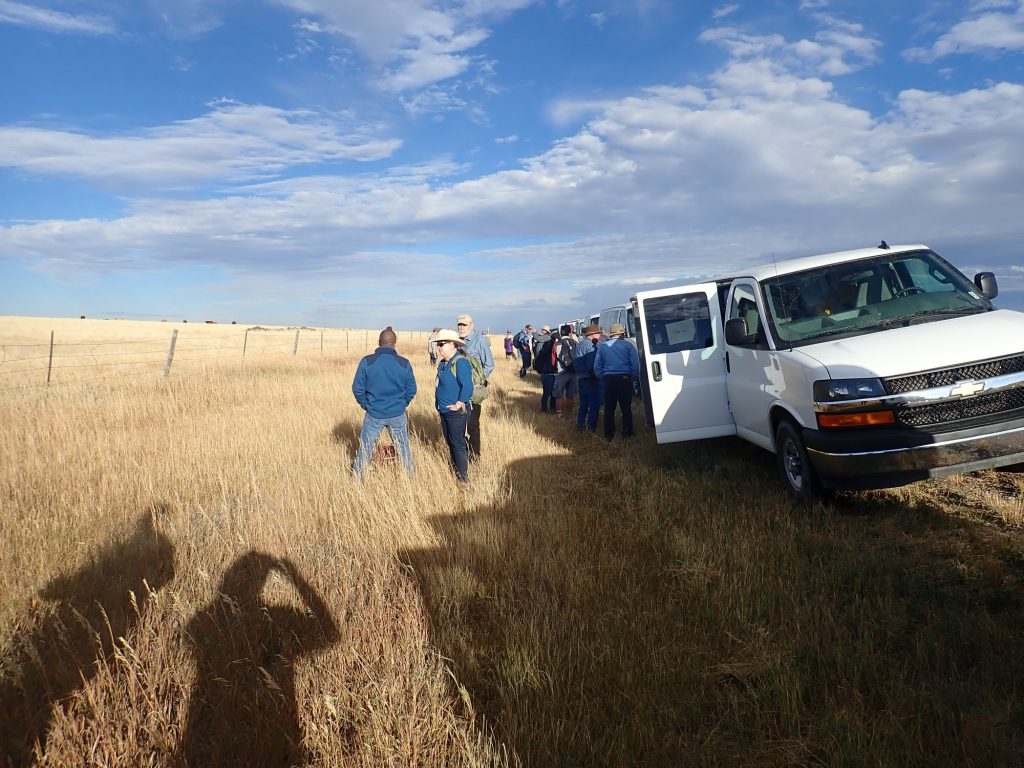
Johnson Mesa is capped by the largest flow of the Raton phase, the first phase of the Raton-Clayton field. Most of the higher mesas in the Raton area are capped with Raton phase flows, an excellent example of inverted topography. Lava that pooled in a low areas produced a hard cap that protected the sediments below while surrounding sediments were eroded away, producing a high mesa where there was once a valley.
To the south is the heart of the volcanic field.
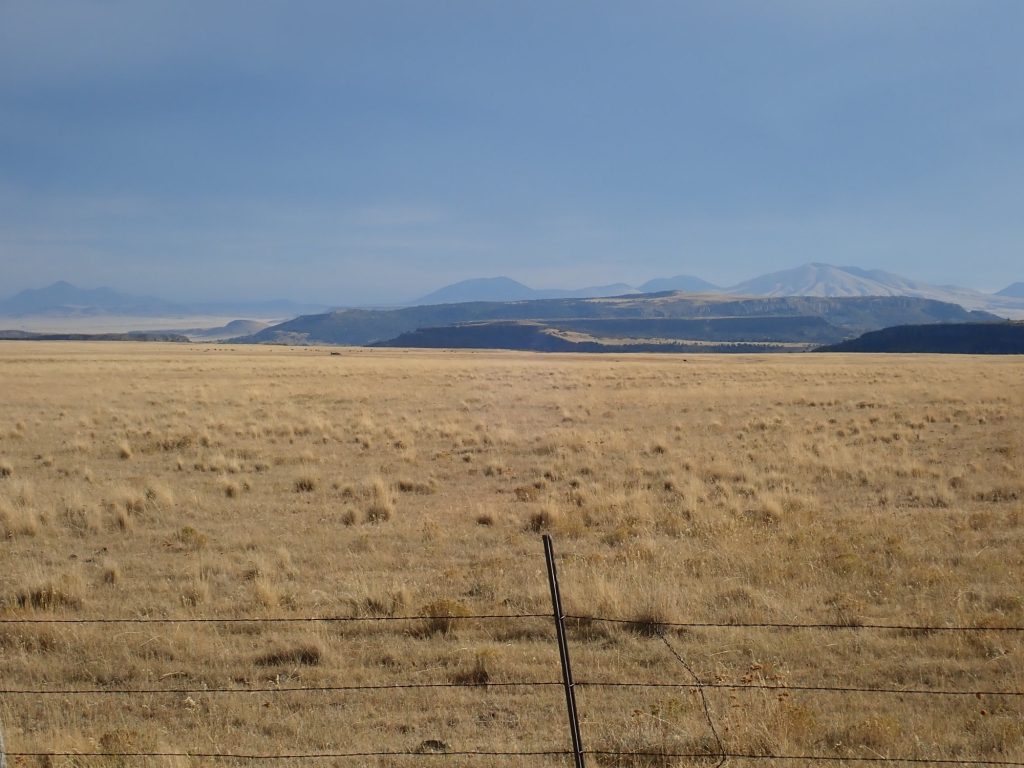
At left in the distance is Palo Blanco mountain; at center is Pine Butte East; and at right is Laughlin Peak. All are underlain by Red Mountain Dacite, a Raton phase rock that is much richer in silica than the basalts and basanites underlying the mesa tops. I discussed bimodal volcanism of this kind in the previous post.
Red Mountain. By an astounding coincidence, this is underlain by Red Mountain Dacite.
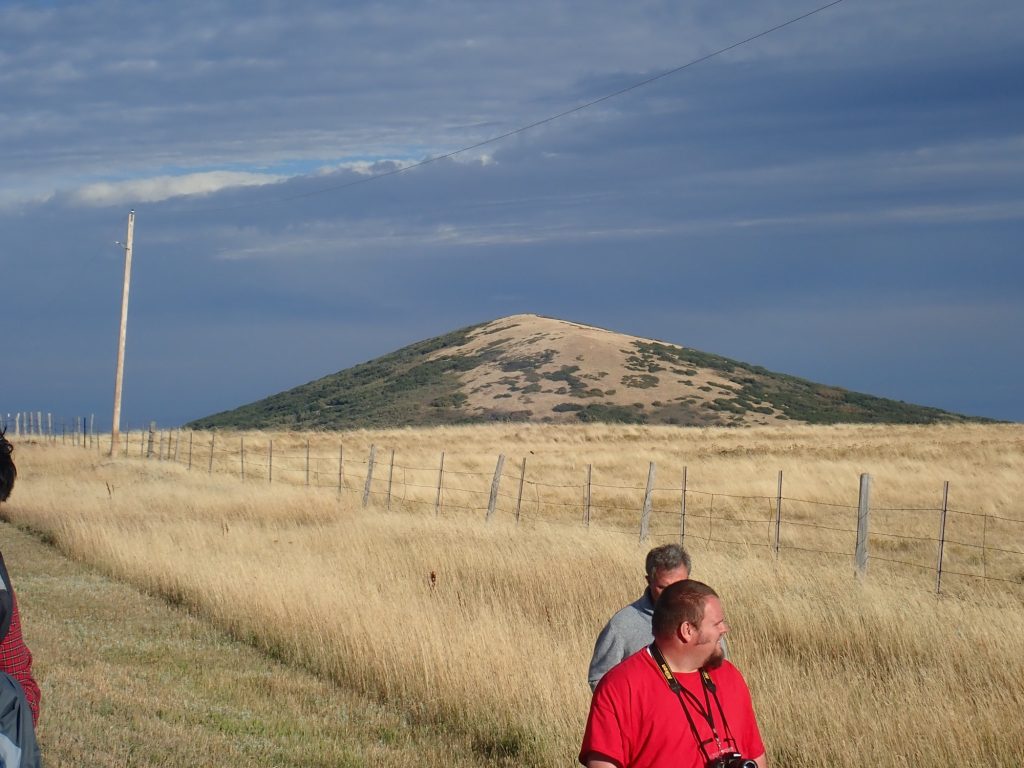
To the north, Dale Mountain.
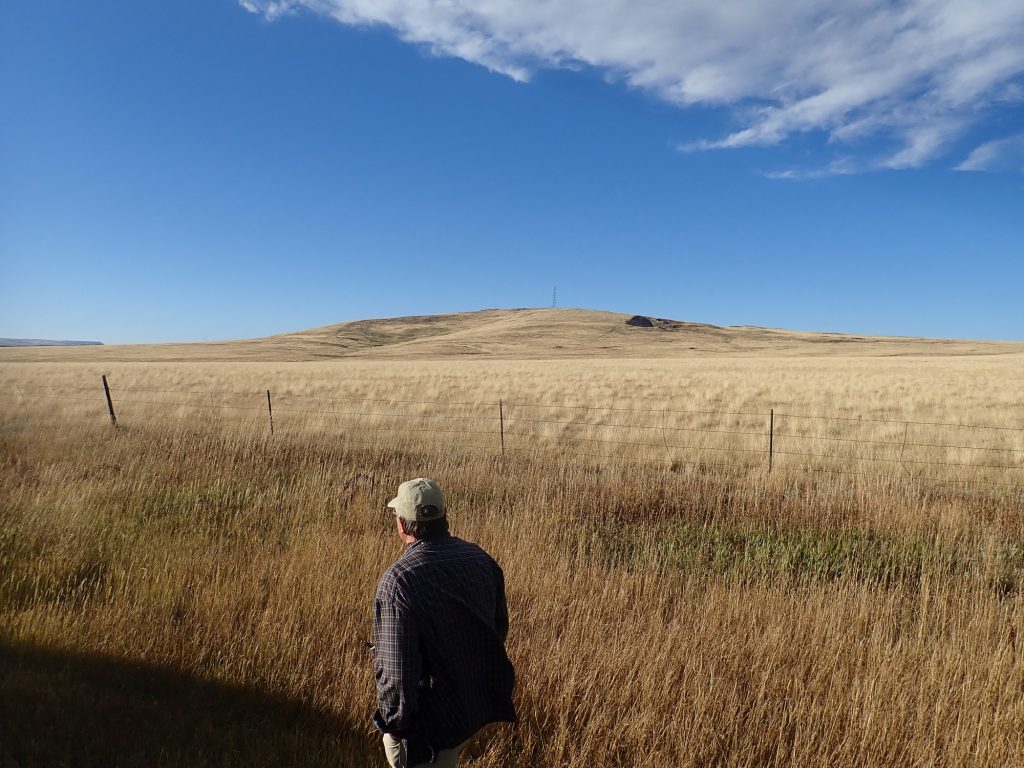
The much gentler slopes of this eruptive center reflect its composition of basanite, which is much less viscous than dacite.
Matt Zimmerer and Kate Zeigler making a presentation.
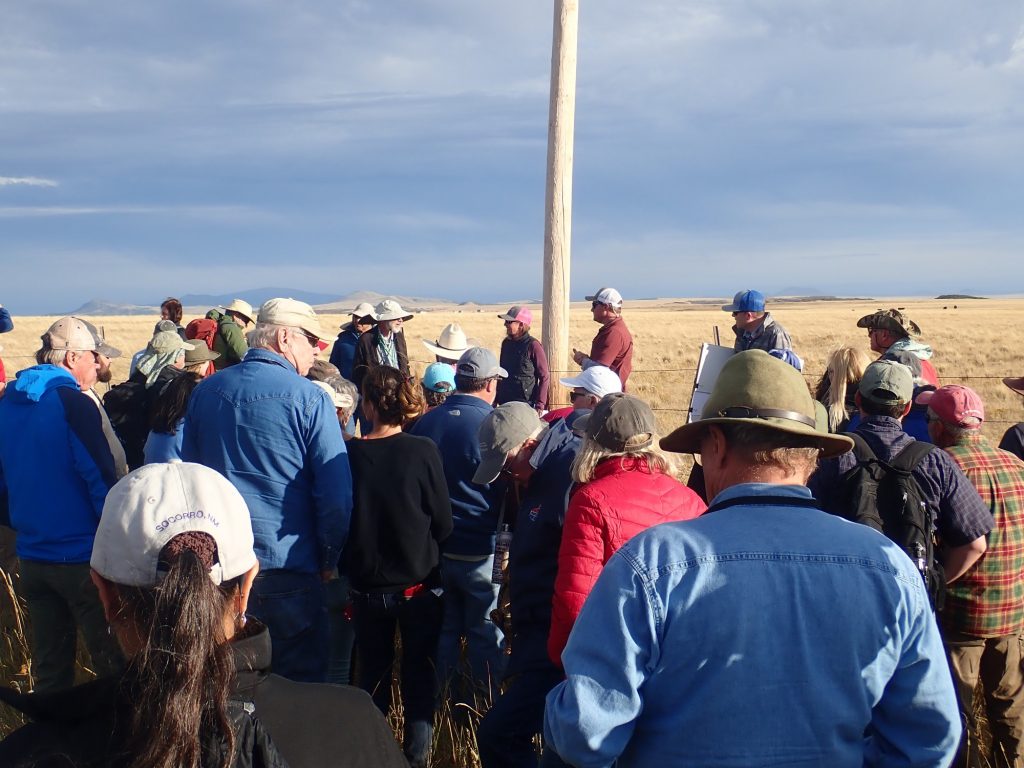
Kate Zeigler goes over the stratigraphy of the area, then discusses her work on ground water in the Clayton area. It turns out that what the local farmer believed was caprock of the Ogallala Formation, a first-rate aquifer, is actually Dakota Sandstone in most places. Ogalalla Formation is found only in a few places near the Texas border, where it fills paleocanyons in the subsurface. And this means that the ground water supply is much more limited than the farmers had believed. The prognosis for agriculture in the Clayton area is not terrific.
Then off to the Folsom Museum for an evening barbeque.
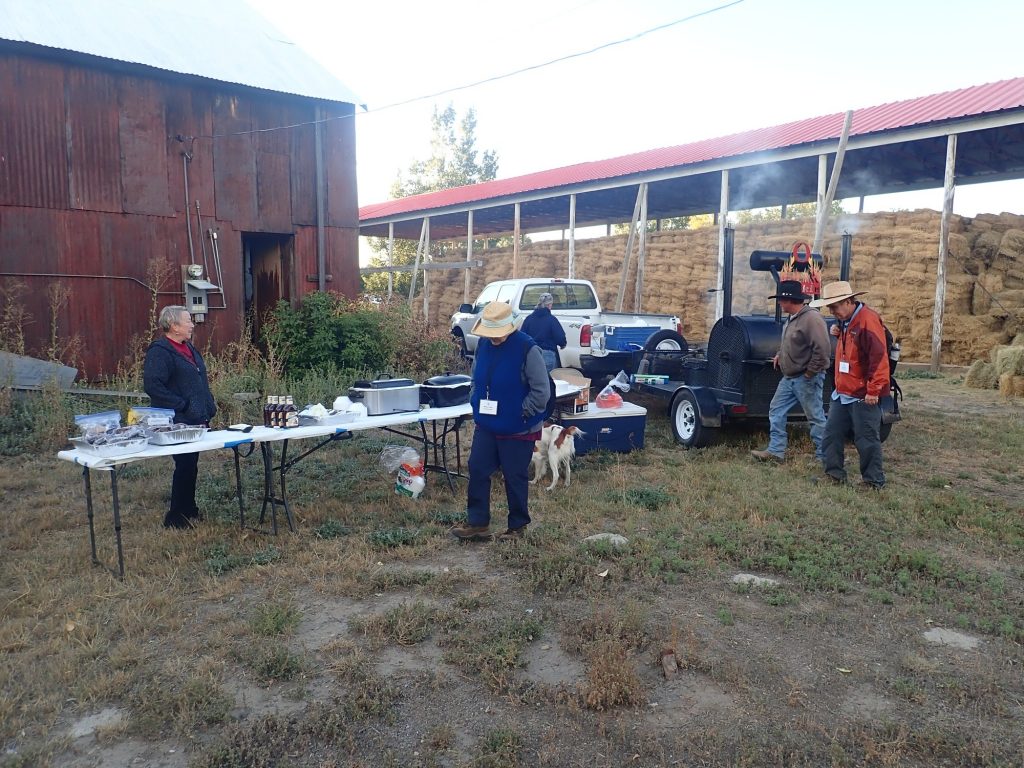
Barbeque beef brisket, baked beans, and coleslaw. Excellent.
Gary gives an undergraduate participant at the conference some sage advice on making it through school. It involves a certain amount of boasting about his daughters’ academic success, for which I hardly blame him.
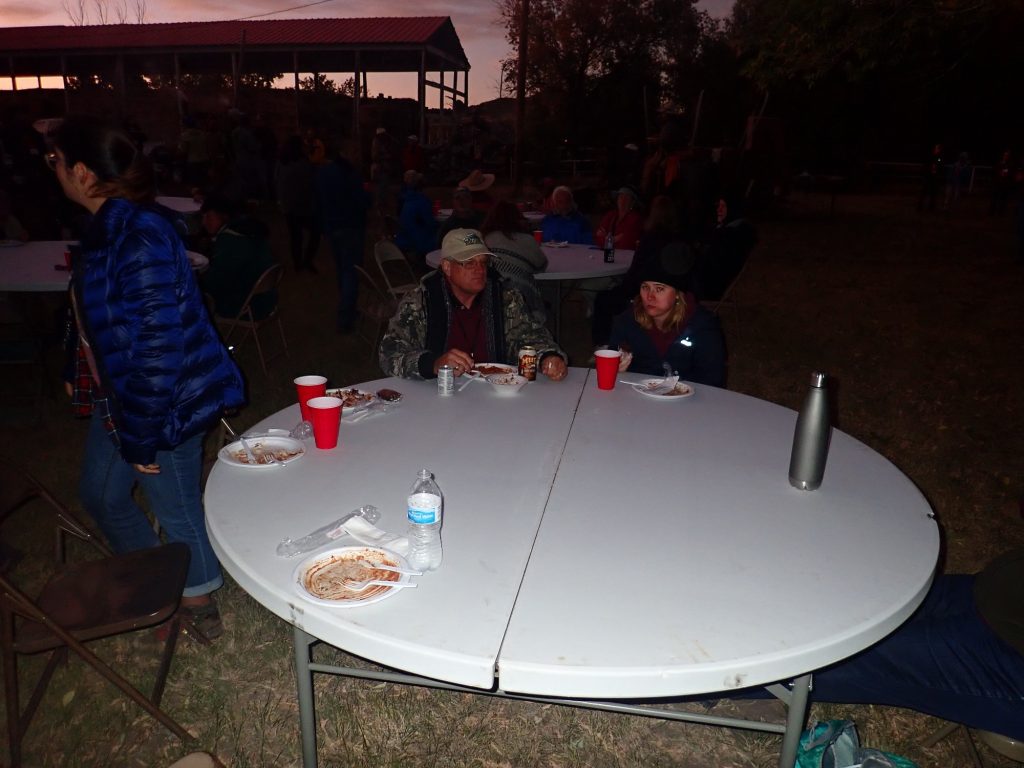
Then back to our camp for the night.