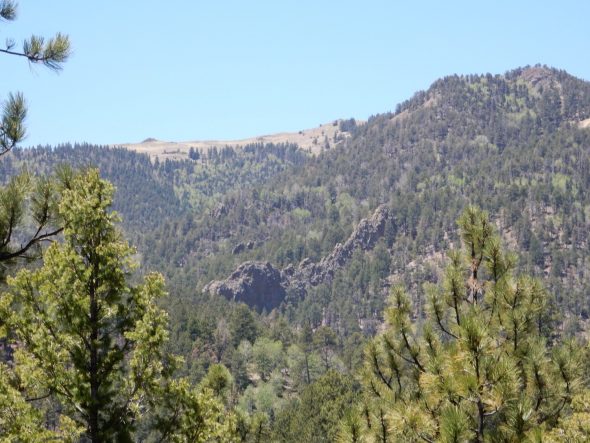
Wanderlusting Mount Taylor, Day 3
It is a beautiful day, without a cloud in the sky. We rendezvous once more to organize car pools.
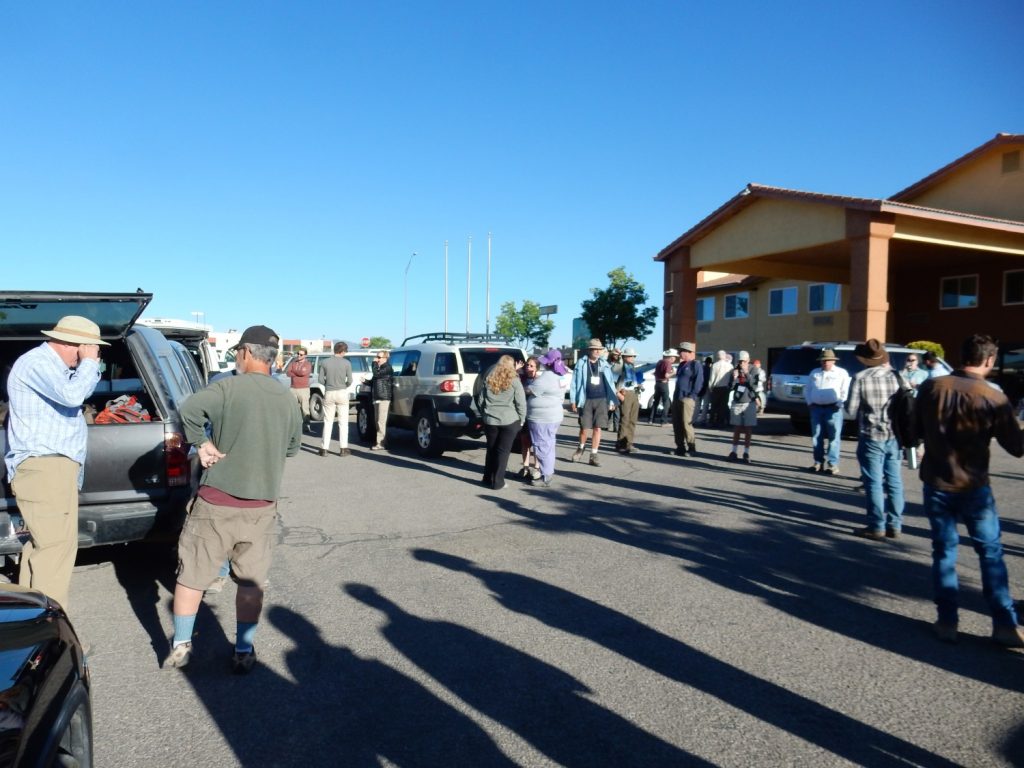
Today we will head into Mount Taylor itself, to the Amphitheater. This looks a little like, but is probably not, a large summit caldera. (More about that later.) We drive east along Old Route 66, then turn north on Water Canyon Road. Ahead is Picacho Peak.
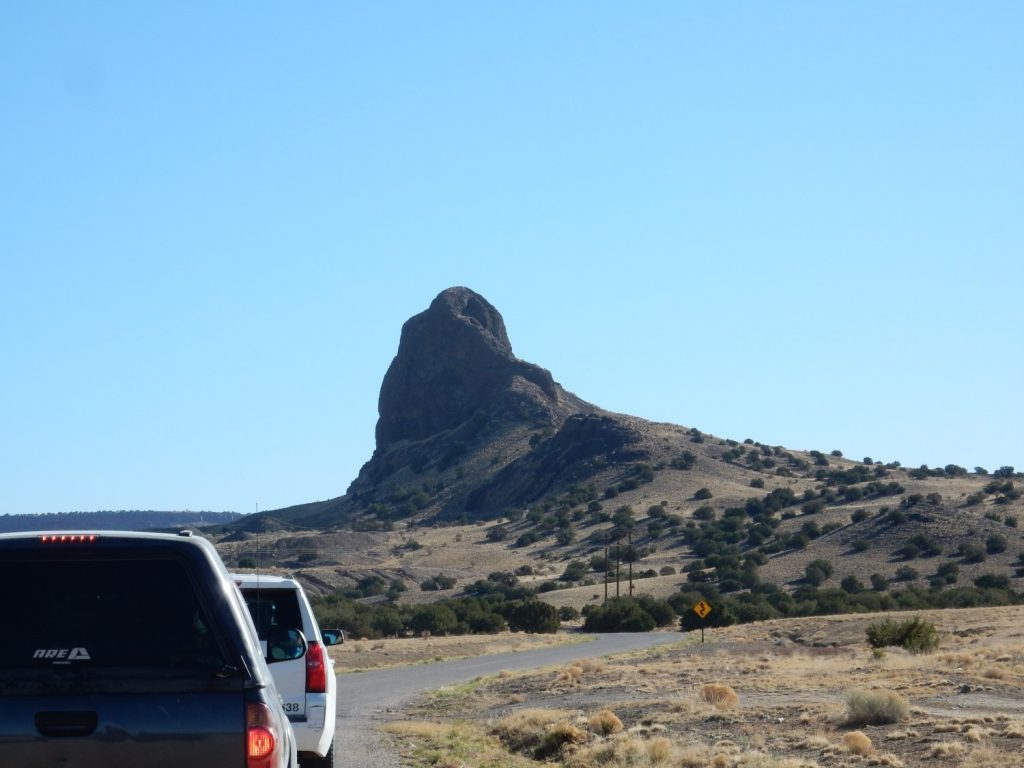
This is a thick dike of olivine basanite, dated at about 4.49 million years old. That makes Picacho Peak part of the earliest and most alkaline volcanism in this area.
I’m going to unpack this fairly carefully, because you can’t talk intelligently about volcanism without saying something about volcanic rock chemistry. Magma, the mixture of molten rock, crystals, and gases that erupts at the surface as lava, is characterized more than anything else by its silica content. Silica (silicon dioxide) has a ferocious tendency to polymerize, forming chains, sheets, or clumps of silicon ions joined by oxygen ions. Metal ions, such as iron, magnesium, or calcium, strongly reduce this tendency towards polymerization. The polymerized silica in high-silica magma makes the magma extremely viscous, while low-silica magma is much less viscous. For example, rhyolite, the most silica-rich common volcanic rock, has a silica content in excess of 69%, and its viscosity is roughly that of cold roofing tar. It can barely make it out of the ground in this state, and so it tends to erupt explosively, as the gases dissolved in the magma bubble out and cause the magma to essentially disintegrate into a cloud of red-hot gas and microscopic shards of half-melted volcanic glass. At the other end of the spectrum, basalt is the least silica-rich common volcanic rock, with a silica content of around 49%, and it has roughly the viscosity of ketchup. This allows basalt to flow considerable distances as lava flows. In between are andesite, with a silica content of about 60% and a viscosity close to that of smooth peanut butter, and dacite, with a silica content of around 66% and a viscosity close to that of Silly Putty. These intermediate lavas can flow, but only a relatively short distance, so they form stratovolcanoes with their classical cone shape. Mount Taylor is a stratovolcano, which should tell you right off that it was formed mostly from intermediate lavas.
Basanite is not a common volcanic rock. It has even less silica than basalt, around 44%, and has an elevated content of alkali metal oxides, around 7% sodium and potassium oxides. Its low silica and high alkali metal content means that it is silica undersaturated; it includes minerals that form only when silica content is low. The most important of these in basanite are olivine and nepheline. Olivine is a magnesium-iron silicate which, if there had been more silica available, would have been transformed to pyroxene, a more silica-rich iron-magnesium silicate that is an important mineral in basalt. Nepheline is a sodium-potassium aluminum silicate which, if there had been more silica available, would have been transformed to feldspar, the most common mineral in most igneous rocks. Calling this rock olivine basanite strikes me as slightly redundant; all basanite contains olivine, by definition. I think our geologists meant that the rock contains small olivine phenocrysts, larger than average crystals, that stand out in the otherwise very fine-grained rock.
Most magma is thought to start out as low-silica magma formed in the upper mantle. How alkaline this is depends on how much magma is extracted from the rock. When mantle rock first begins to melt, the most alkaline minerals melt first. As more magma is extracted from the rock, the alkalinity drops. Mount Taylor is thus thought to have formed from mantle rock that has not had much magma previously extracted from it. The original low-silica magma, called a primitive magma, takes a long time to rise to the surface; along the way, it may be contaminated by more silica-rich crust rock, and some low-silica minerals may crystallize out of it, increasing its silica content. Geologists say that the magma becomes more evolved. This is likely the main source of intermediate magmas. The most silica-rich magmas, such as rhyolite magmas, likely formed from melting of crustal rock from the heat of low-silica magma that never reached the surface. The magma stagnated instead deeper in the crust.
We reach the entrance to the Mount Taylor Ranch, now owned by Laguna Pueblo, which is graciously hosting us on this trip. We are reminded how dry the conditions are and how careful we need to be about fire danger.
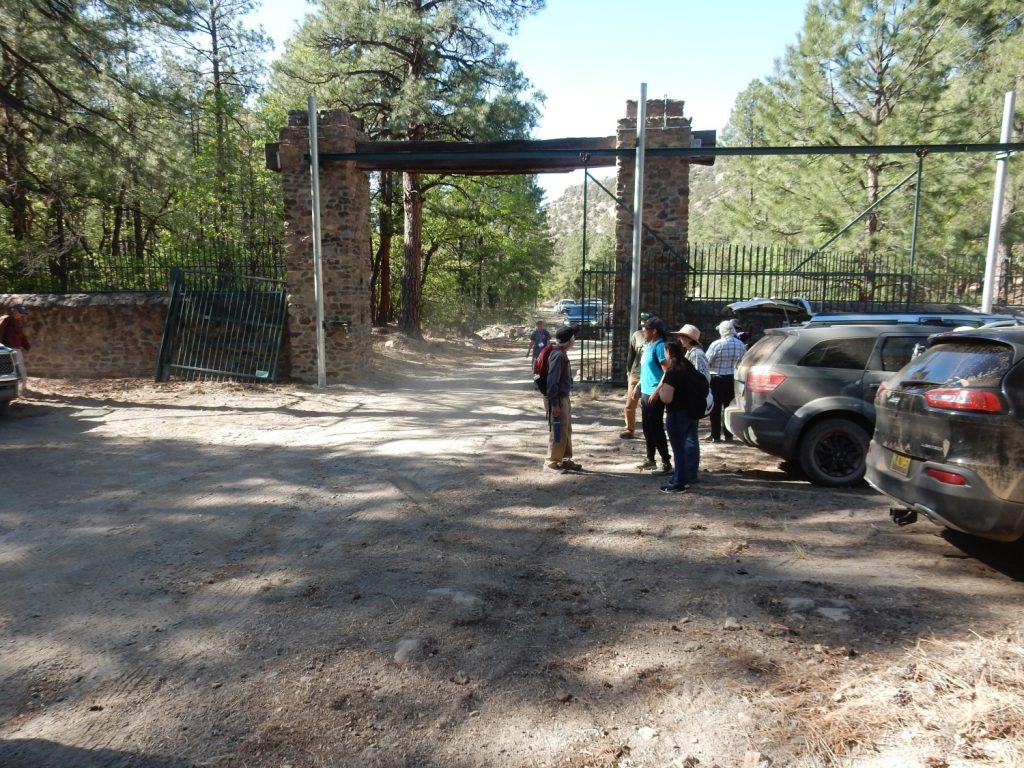
We are driving up Water Canyon into the center of the volcano. This canyon is an erosional feature; the reasons for such extensive erosion of hte heart of the volcano will be explained presently.
We gather, and the posters come out again.
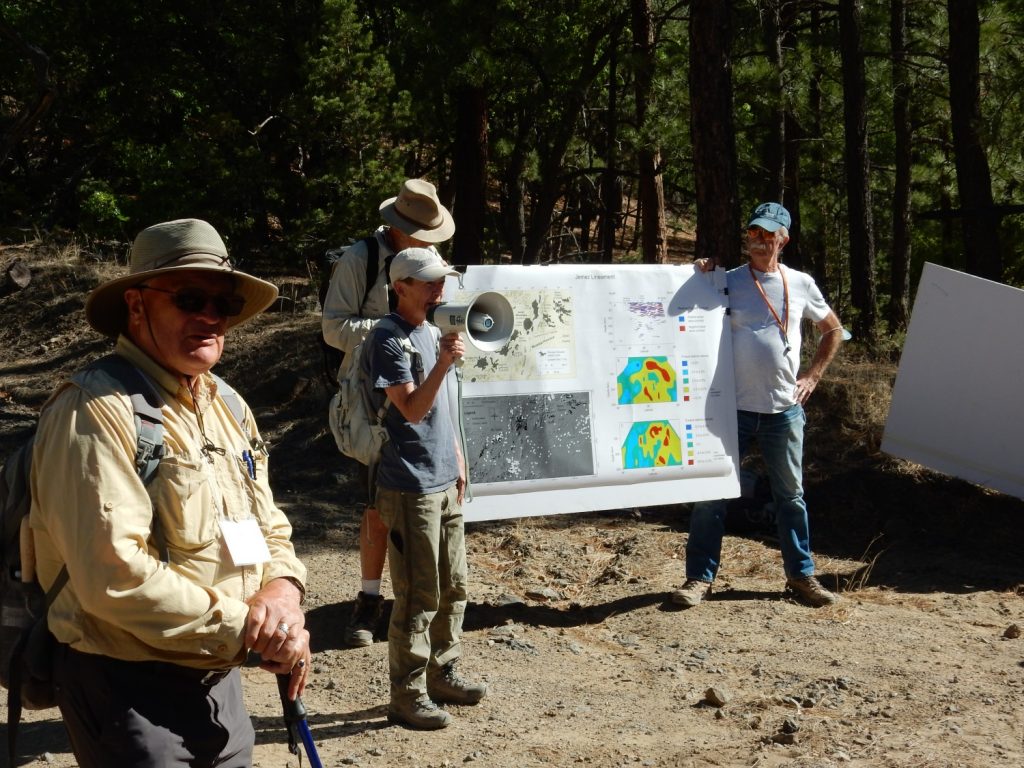
Fraser Goff and Shari Kelley discuss the Jemez Lineament, a chain of volcanic fields stretching from southeastern Arizona through the Mount Taylor area and on through the Jemez Mountains to the Raton-Clayton volcanic field in northeastern New Mexico. This is a slightly mysterious feature of the Earth’s crust, though we have some good ideas what it is about.
There is no systematic progression in age along the lineament, so it is not a hot-spot trace, like the Snake River Plain in Idaho. Hot-spot traces form when the continent drifts across a hot upwelling from deeper parts of the earth. (However, there are local age trends within individual volcanic fields showing continental drift.) There are not obvious structural features,such as systems of faults, suggesting that the Jemez Lineament is incipient rifting of the Earth’s crust. The geophysical evidence suggests this is an ancient suture between different sections of crust, dating clear back to 1.8 billion years ago, when North America first assembled. The suture likely contains rock rich in hydrous minerals that are especially prone to melting to form magma. One of our student scholars from UNM tells me that that the Jemez Lineament “is my life”, which is not unreasonable for a budding geologist thinking about dissertation research. I wish her the very best of luck learning more about this feature, and look forward to seeing her results.
The Mount Taylor map comes out again, and Fraser Goff reviews what is known about the volcano. It was erupted in four episodes stretching over about two and a half million years. The first phase, from 3.72 to 2.91 million years ago, consisted of basanites and some alkali basalts that capped mesas in the area and formed a kind of floor for the volcano. There were also dome eruptions, of rhyolite trachydacite (alkali-rich dacite) that produced some pyroclastic flows. Pyroclastic flows are a form of explosive volcanism, in which fragments of volcanic glass mixed with red-hot gases form a dense mixture that hugs the surface, but can flow for great distances before settling out to form tuff.
The second phase, from 2.9 to 2.75 million years ago, formed much of the stratovolcano. The lavas were mostly trachyandesite, trachydacite, and trachyte, all intermediate- to high-silica lavas with elevated alkali content. This phase ended with voluminous eruptions of “plagioclase basalt” or “big feldspar basalt”, a silica-poor volcanic rock containing large white crystals of plagioclase feldspar.
The third phase, from 2.75 to 2.5 million years ago, came from a central vent that intruded magma as dikes in the surrounding stratovolcano beds. This completed the construction of the edifice but also weakened the central part of the volcano, rendering it susceptible to the erosion that produced the Amphitheater.
The final phase, from 2.5 to 1.26 million years ago, was mostly low-silica magma that erupted from vents on the flanks of the volcano.
We begin our hike up the west canyon wall.
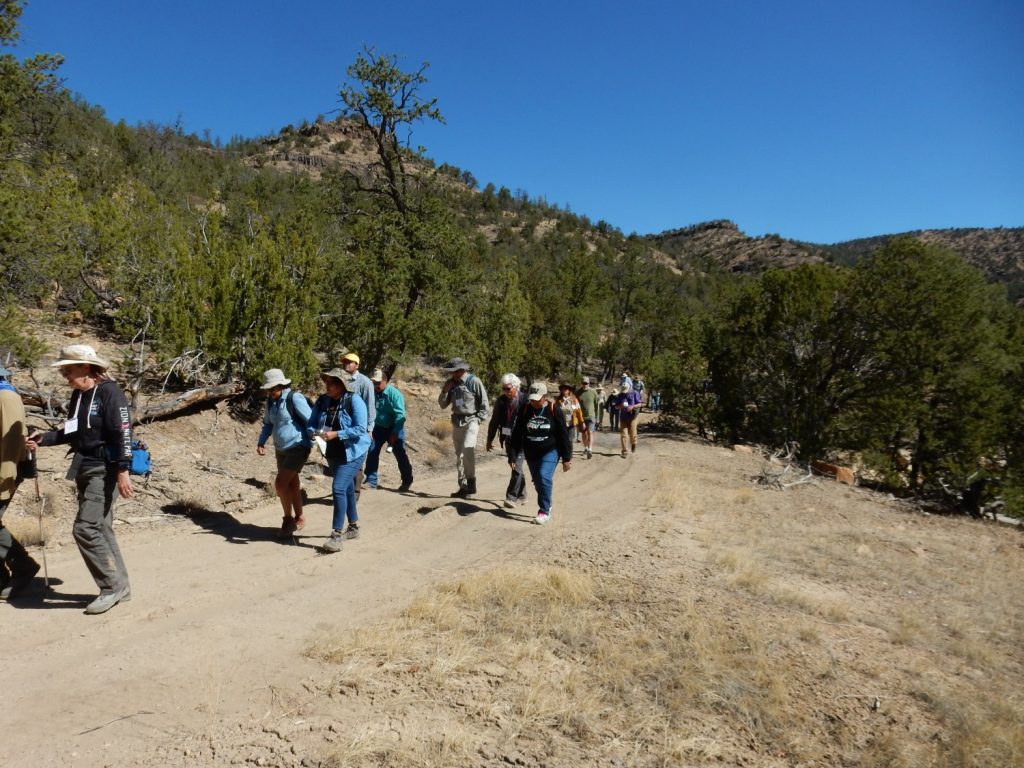
There is a nice view down Water Canyon from here.
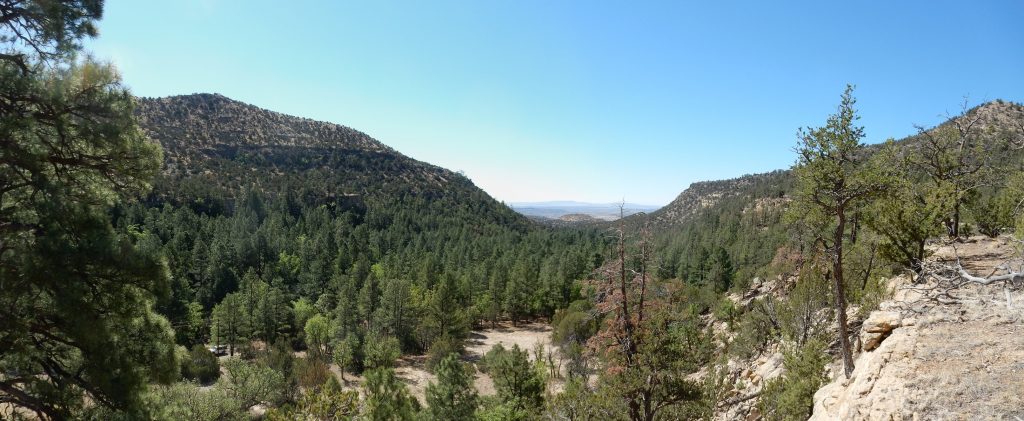
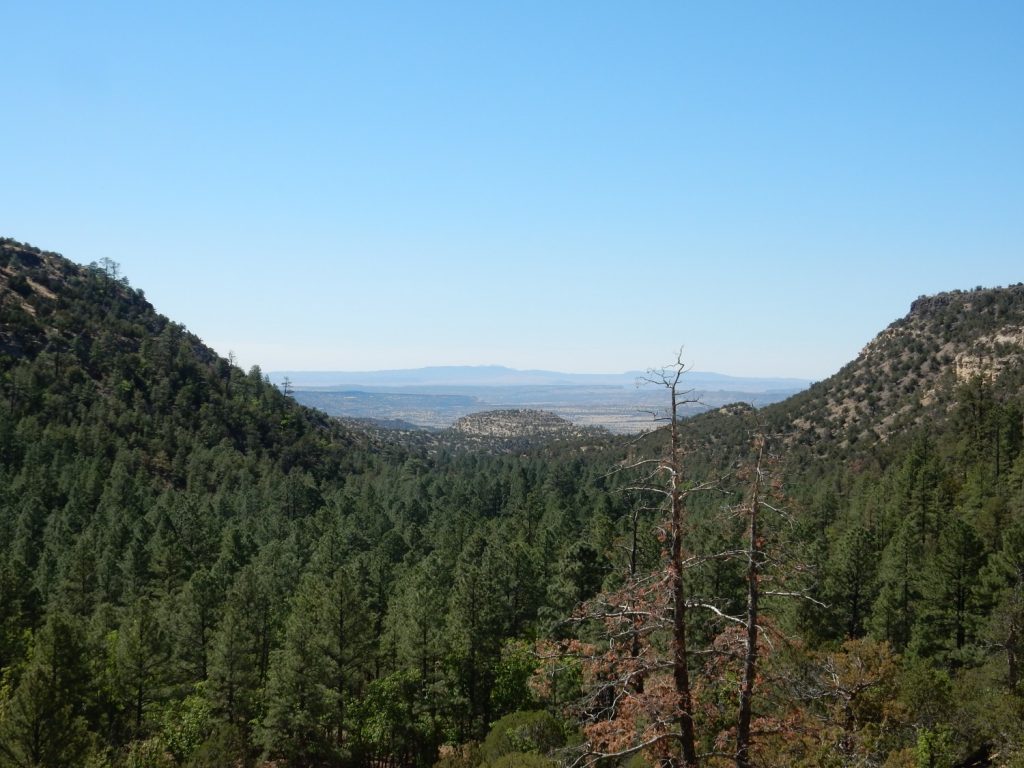
The shelf of sandstone I am standing on for these photographs is the Dalton Sandstone Member of the Crevasse Canyon Formation. This formation underlies the lower slopes of the canyon walls and the mesa at the end of the canyon. The cliff halfway up the left (east) canyon wall is alkali basalt and basanite flows, over which are found the tuff beds of Water Canyon. The lavas are about 3.2 million years old; the tuff ranges from 3.04 to 2.74 million years old. The uppermost parts of the canyon wall are volcaniclastics, beds of rock fragments eroded out of the Amphitheater and carried to the east by debris flows.
We gather again for more discussion of stratigraphy.
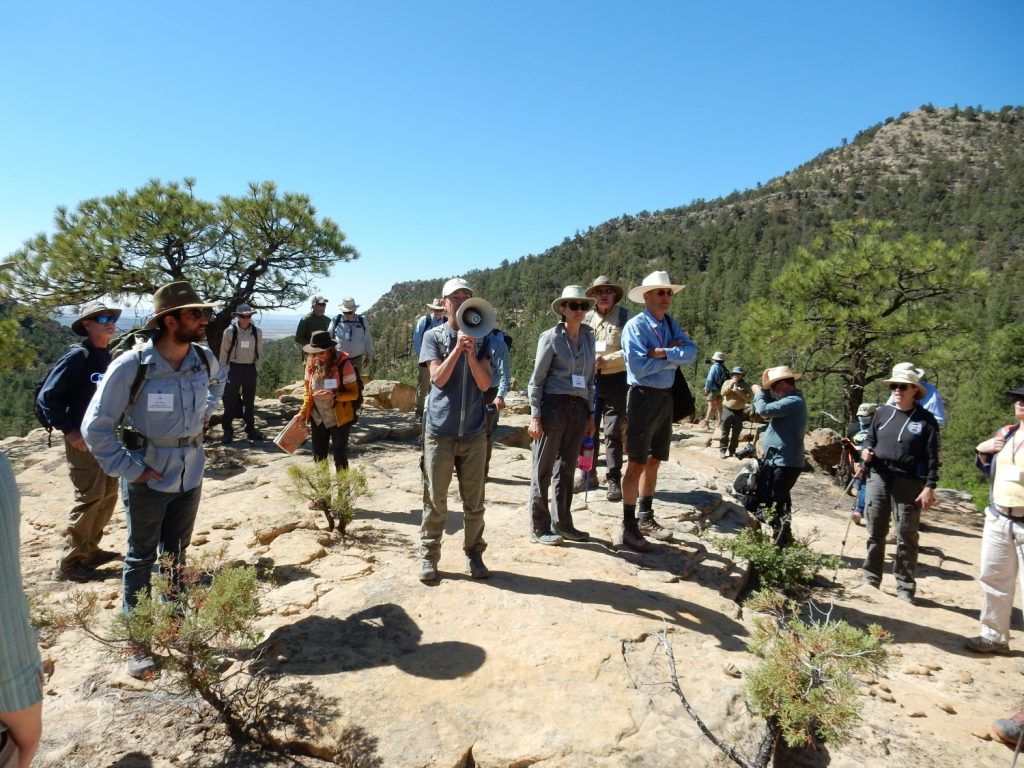
Another view of the east canyon wall showing the beds discussed earlier.
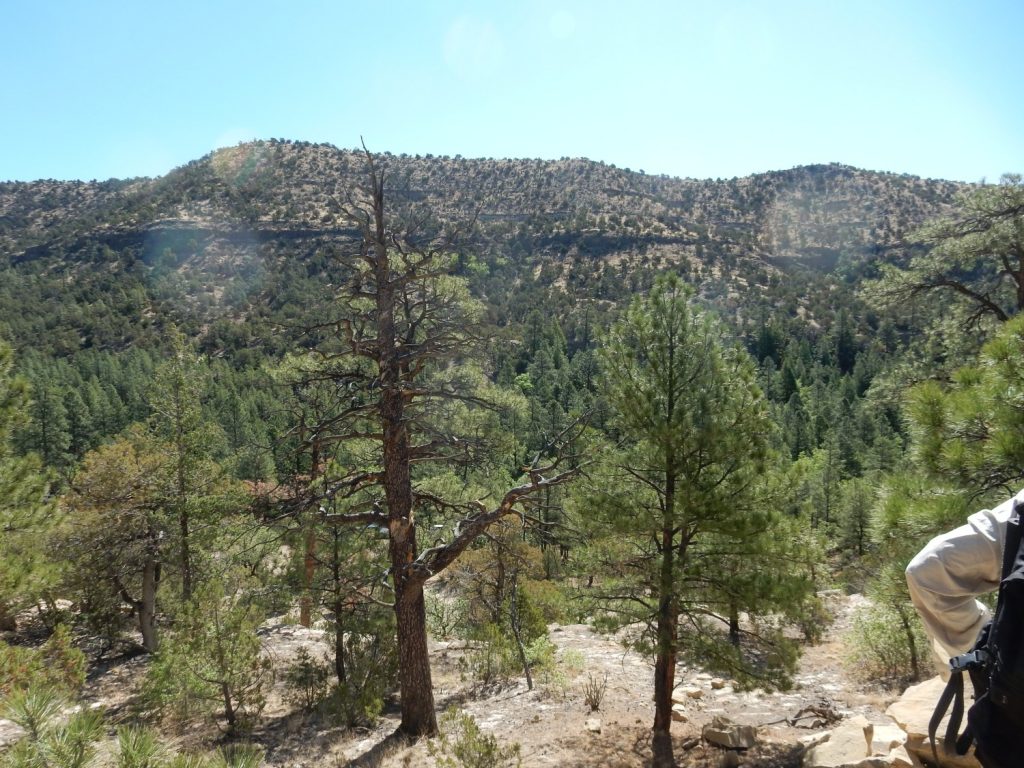
The tuff beds are well exposed to the west.
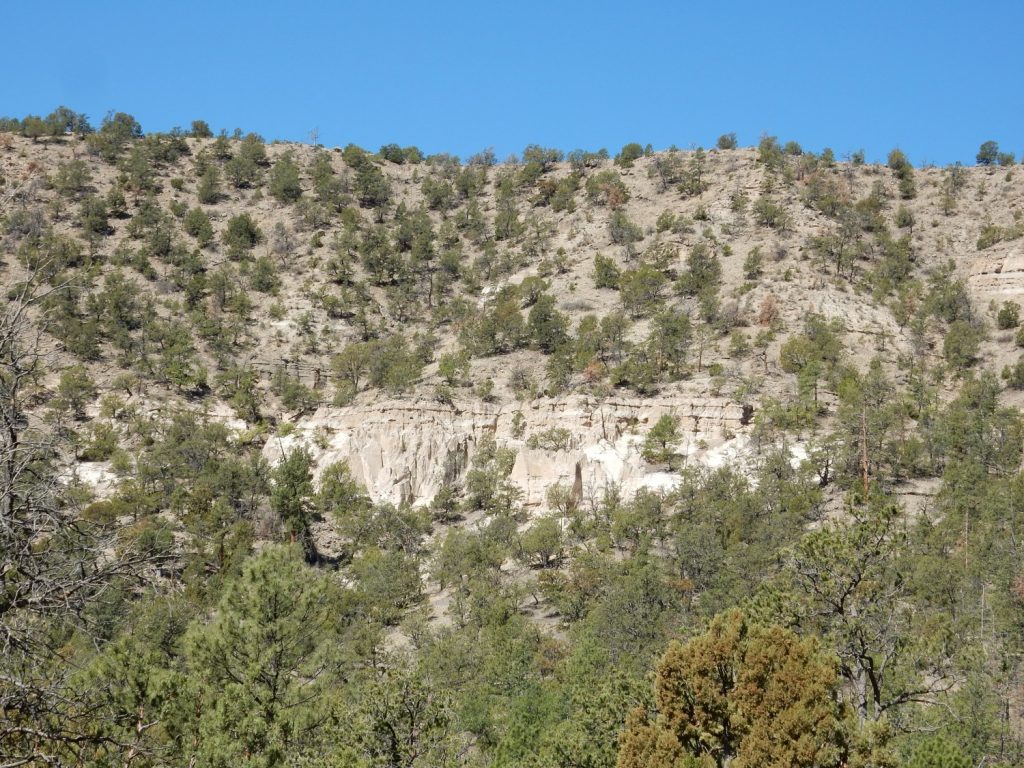
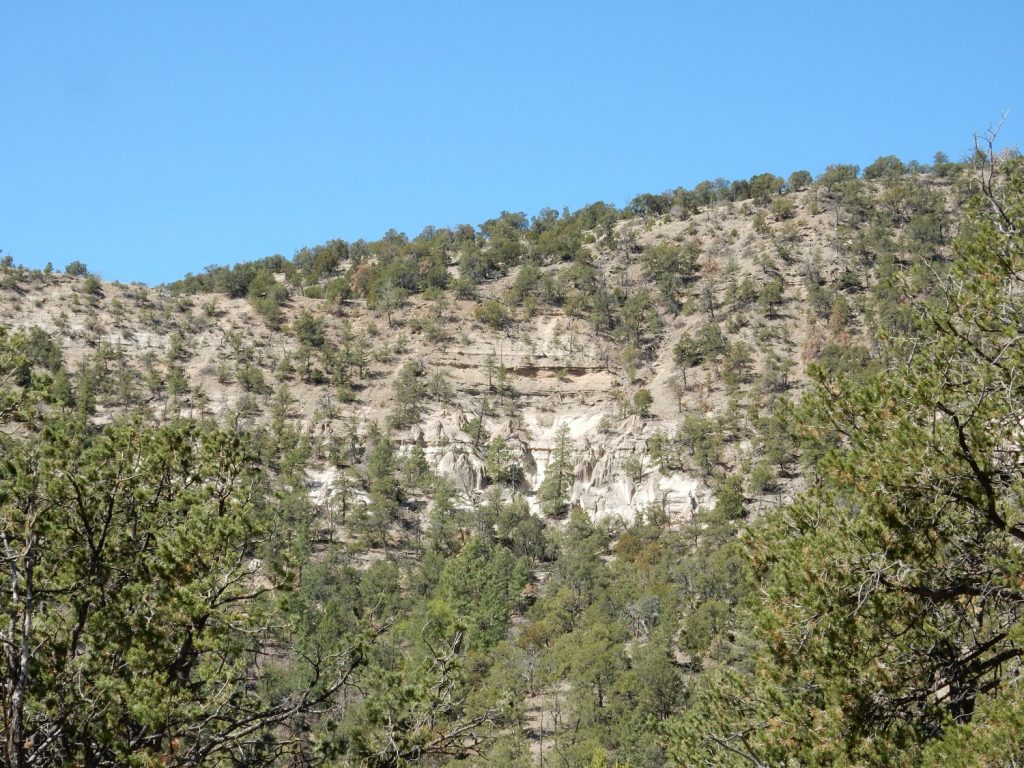
Continuing up the trail.
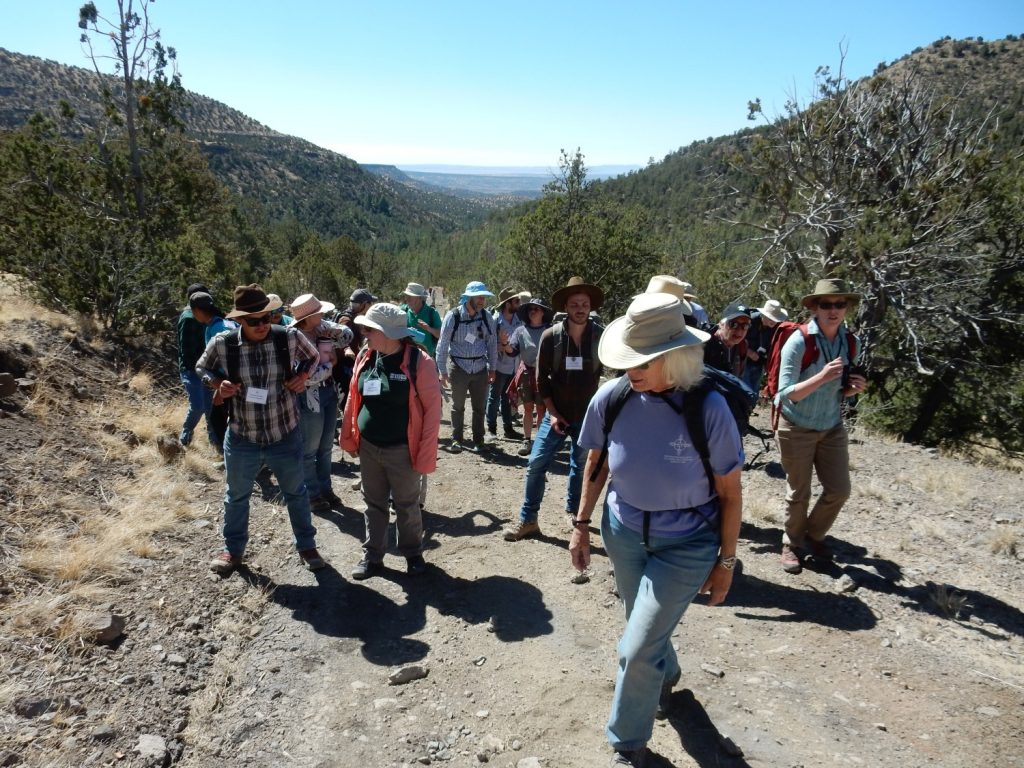
We come to a thin coal bed of the Gibson Coal Member of the Crevasse Canyon Formation, not unlike what we saw at San Mateo Mesa yesterday. However, the overlying layers of Point Lookout Sandstone are missing here.
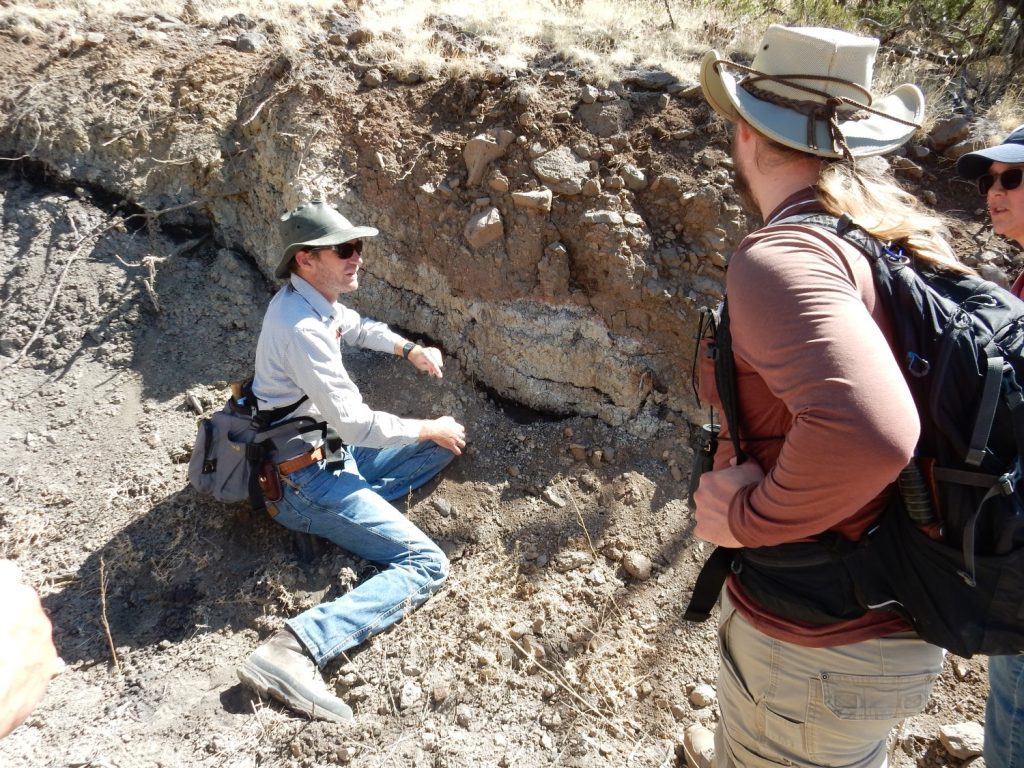
Panorama of the tuff beds and volcaniclastics to the west.
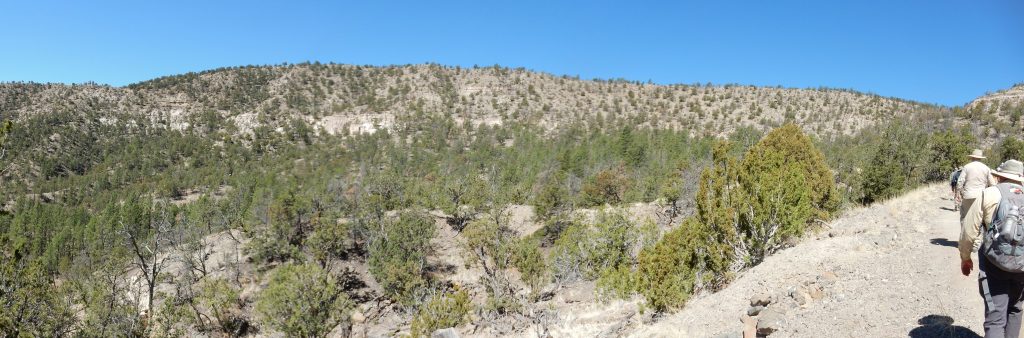
We arrive at a bend in the trail that will be as far as we hike. We are trying to keep to a schedule.
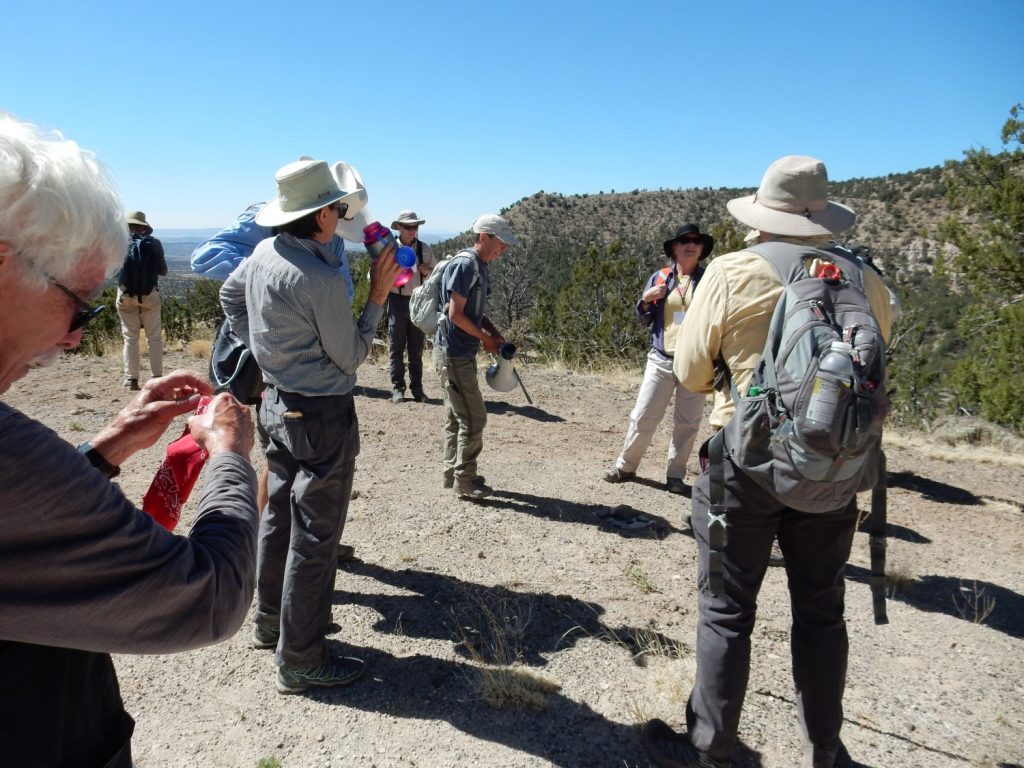
Panorama.
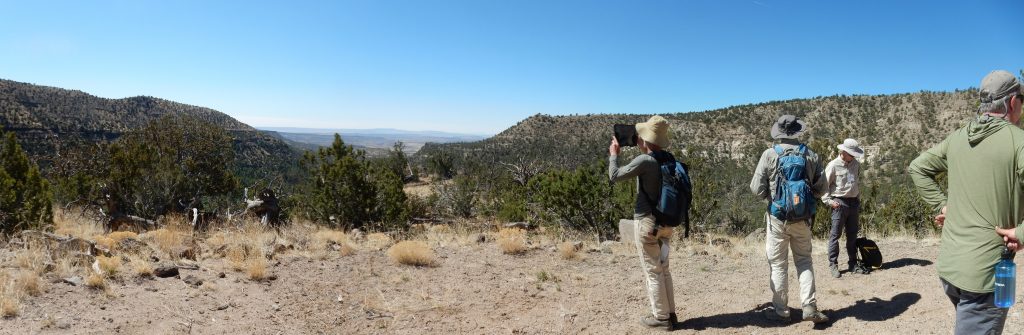
In the far distance, we look over Gallina Mesa at Ladron Peak, far to the south.
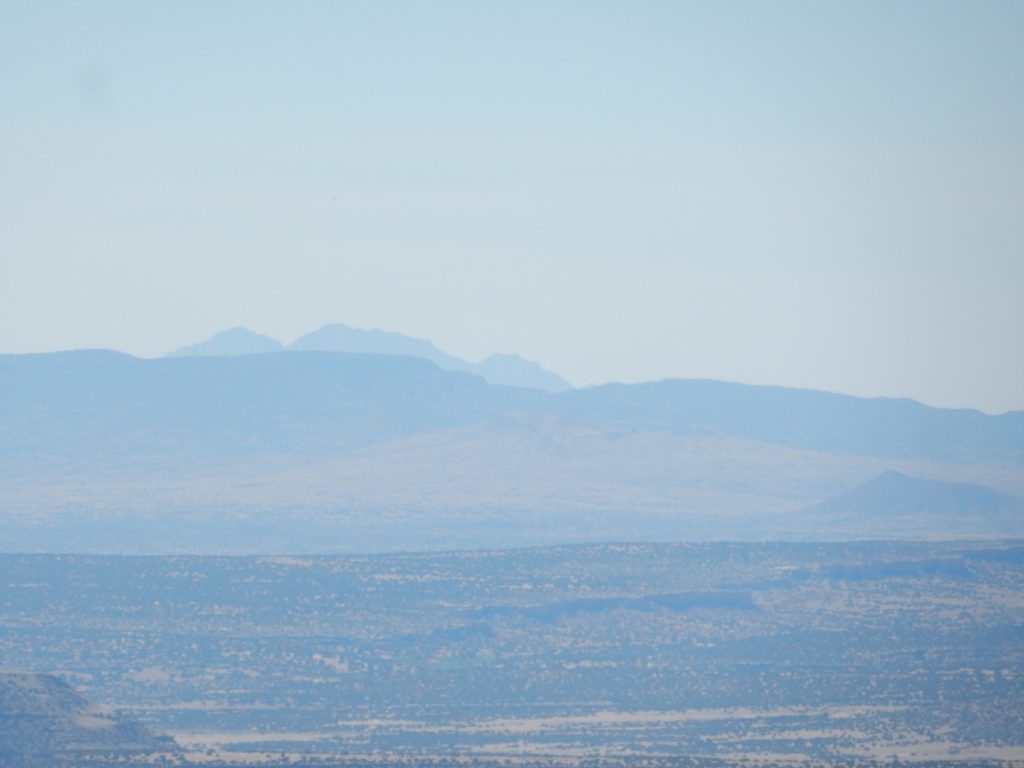
Ladron Peak is not too far from the area chosen for our next conference, in Socorro, though I don’t think a field excursion to the peak itself is in the works.
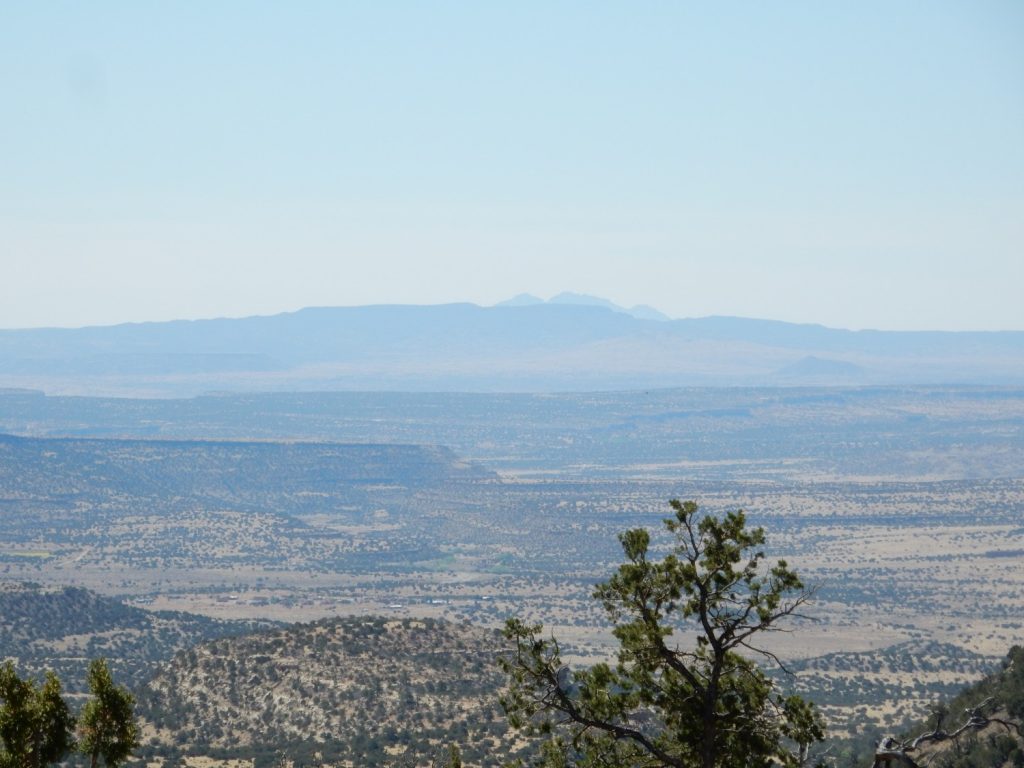
We’ve reached the basanite flow mentioned earlier.
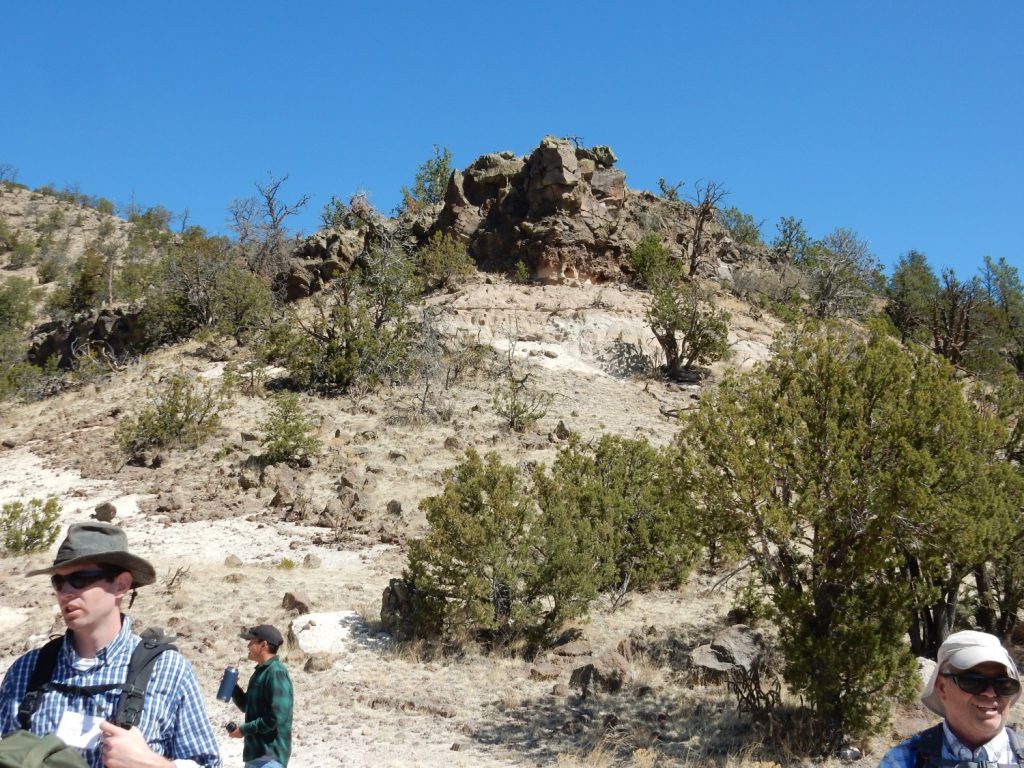
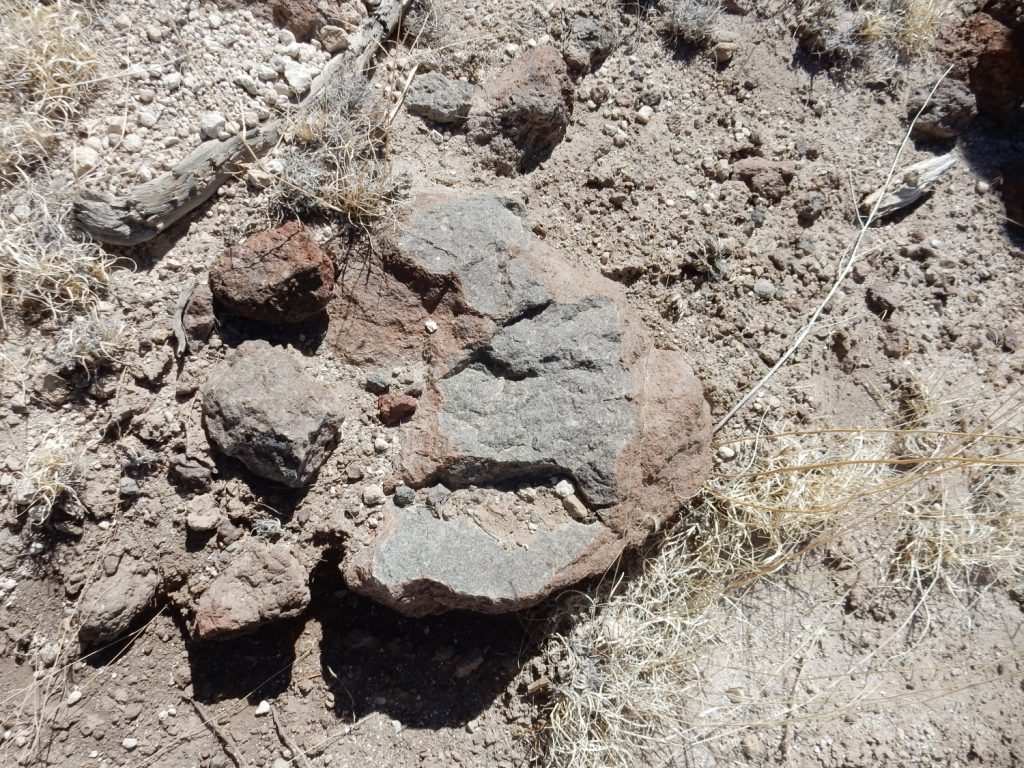
Basanite is typically rich in magnetite (ferrous-ferric oxide), which weathers to a dark red color, as you see in the photograph.
The tuff further up the road:
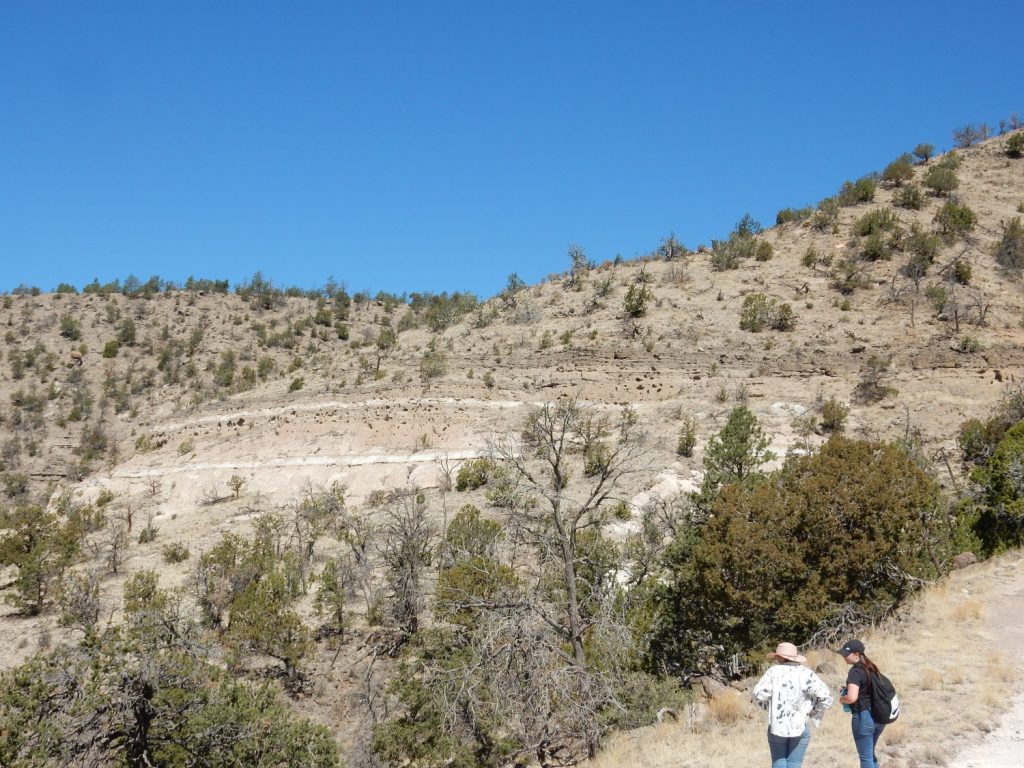
Then it’s time to descend.
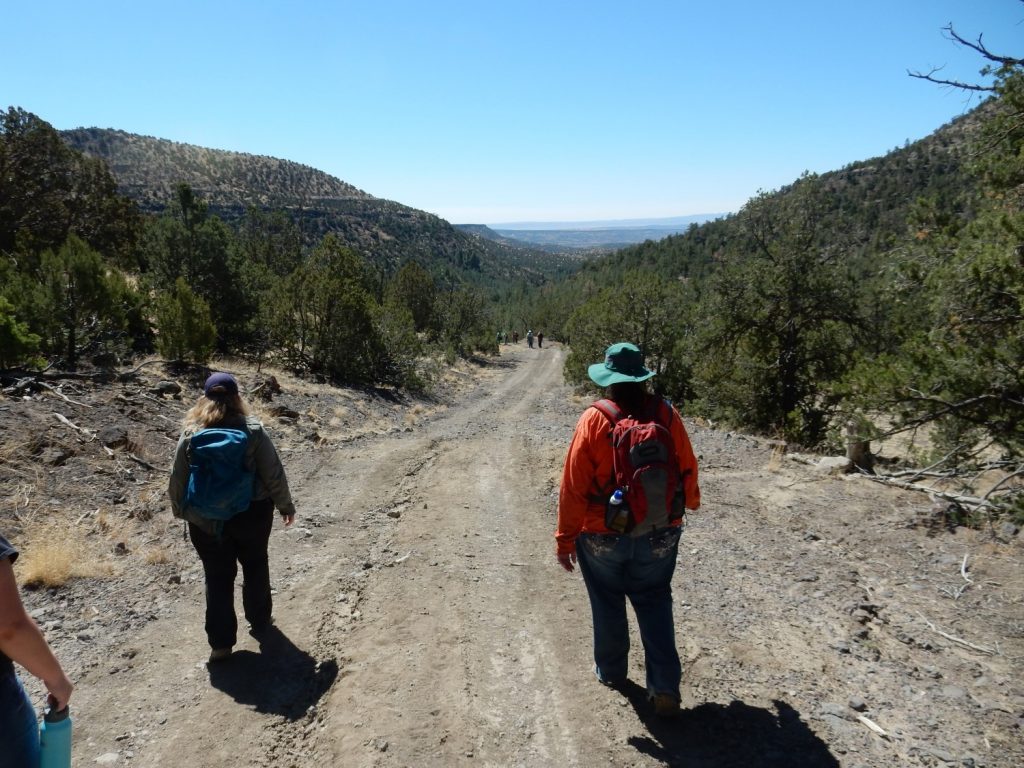
We proceed to the old hunting lodge
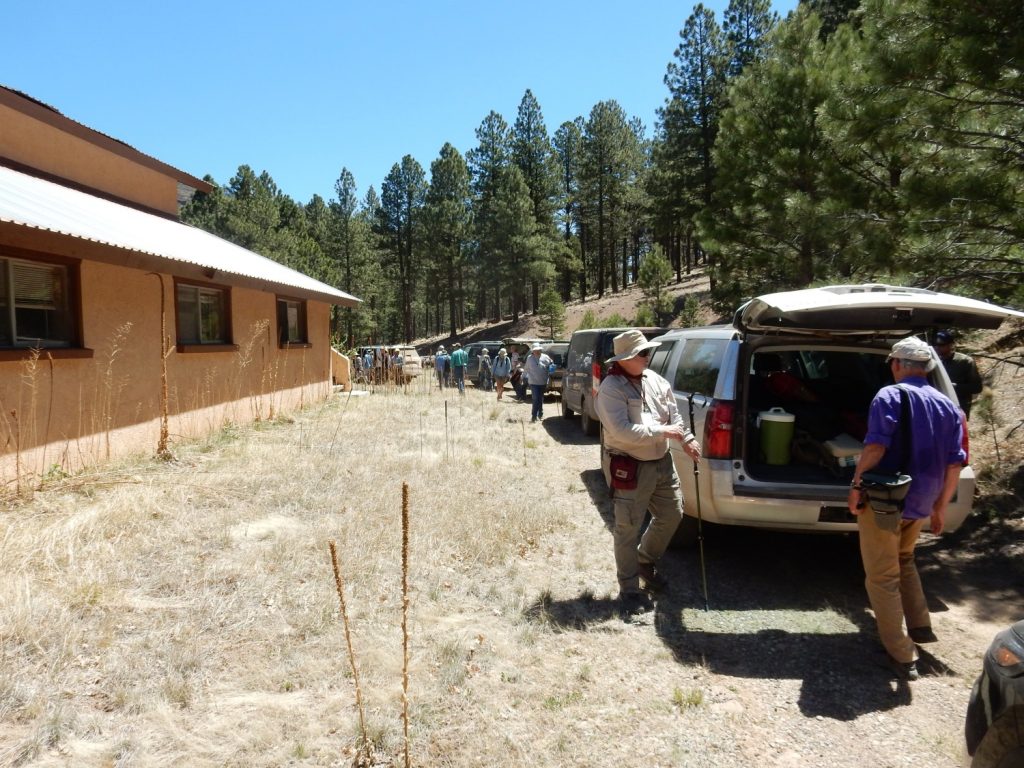
in the eastern part of the Amphitheater. It turns out this is not actually where we want to park, but our cars are soon lined up along a section of road where we will not ignite the grass.
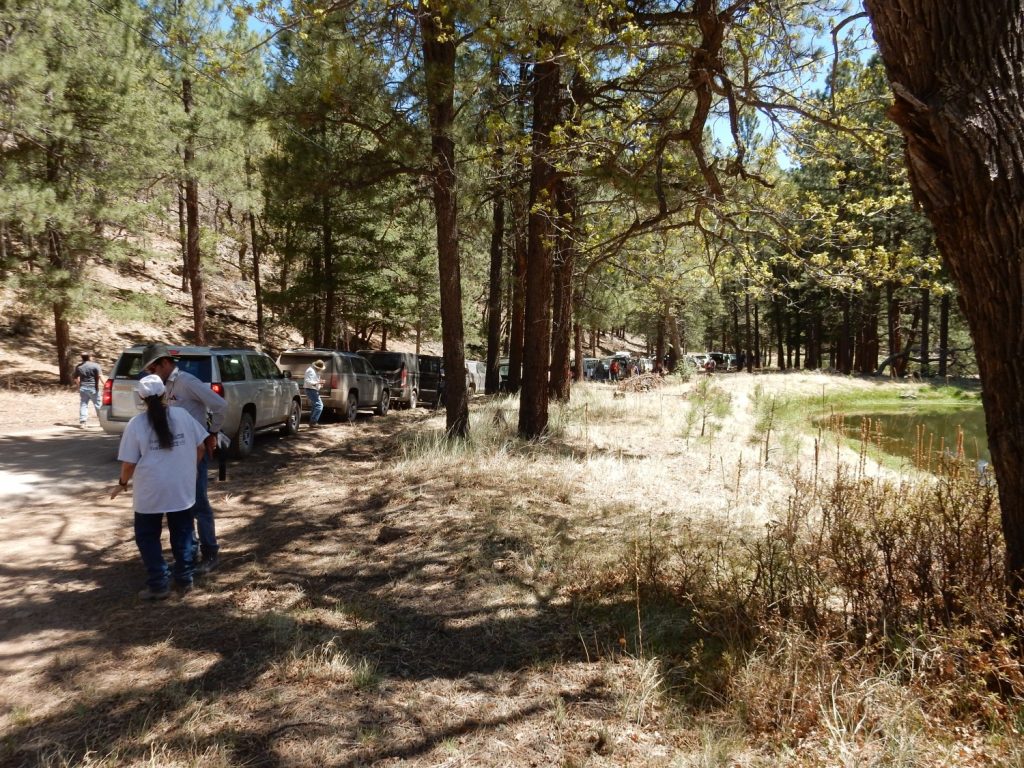
I take in the view to the north.
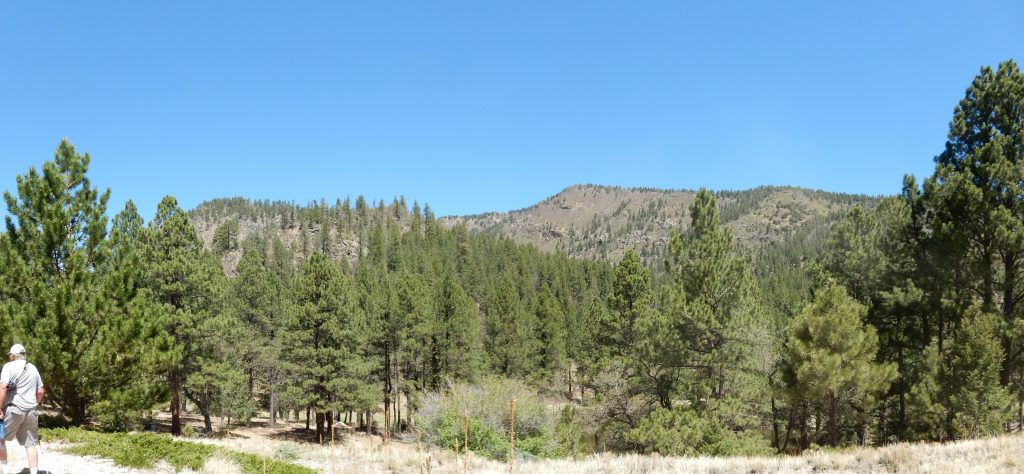
At right is the northeast rim of the Amphitheater. The lowermost part is more amphitheater basanite, hidden behind the trees. Above is a thick section of a rock described as porphyritic-biotite trachydacite, which I believe forms the visible cliffs. This is another alkaline intermediate rock, showing visible phenocrysts of biotite. It’s one of the few units in Mount Taylor that Fraser Goff never got a date on. Above are two flows of the plagioclase “basalt”, the lower actually a trachyandesite and the higher actually trachybasalt. The higher is about 2.76 to 2.79 million years old. This is the flow that caps the second phase of volcanism at Mount Taylor.
The hill at left is actually a plug of gabbro and one of the more surprising features that Fraser and friends mapped here. Gabbro has the same composition as basalt, but is coarsely crystalline due to cooling very slowly underground. The gabbro here contains a few mantle xenoliths. It also hydrothermally altered the rocks around it as it was intruded into them. The surprising thing is its age, just 1.97 million years, placing it firmly in the final phase of volcano building here. The plug likely contributed to weakening the rock that was later eroded away to form the Amphitheater.
We will not visit the plug, which is off the Laguna Pueblo land. (Darn!)
Of course, I know all this only because Fraser Goff explained it all.
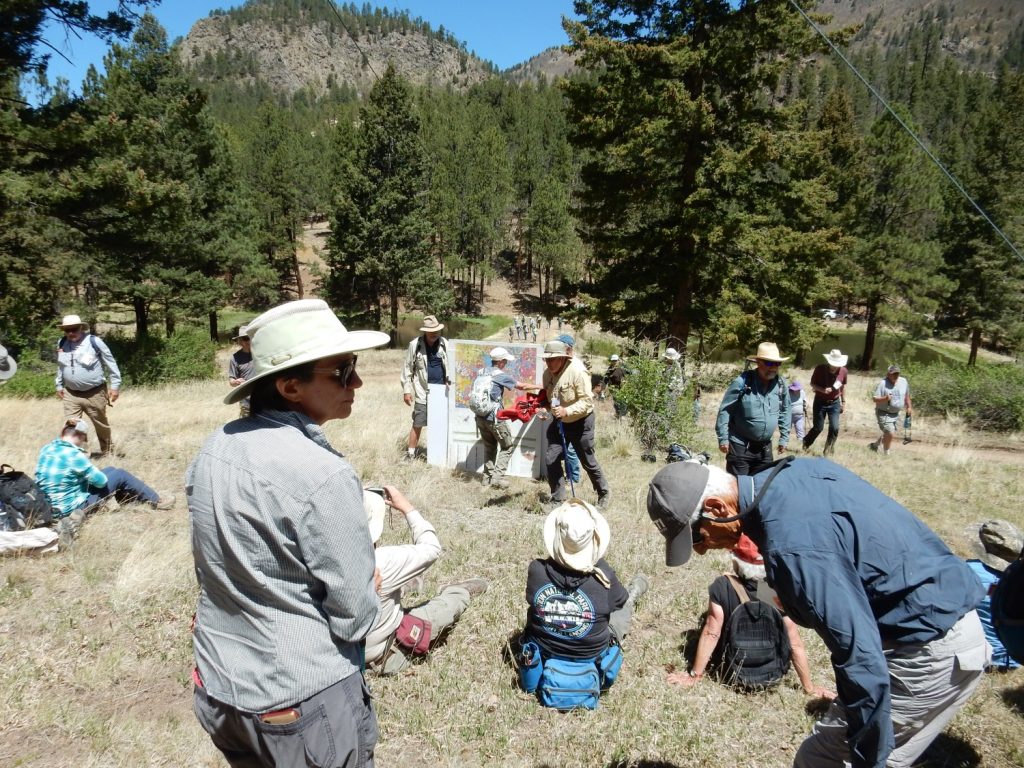
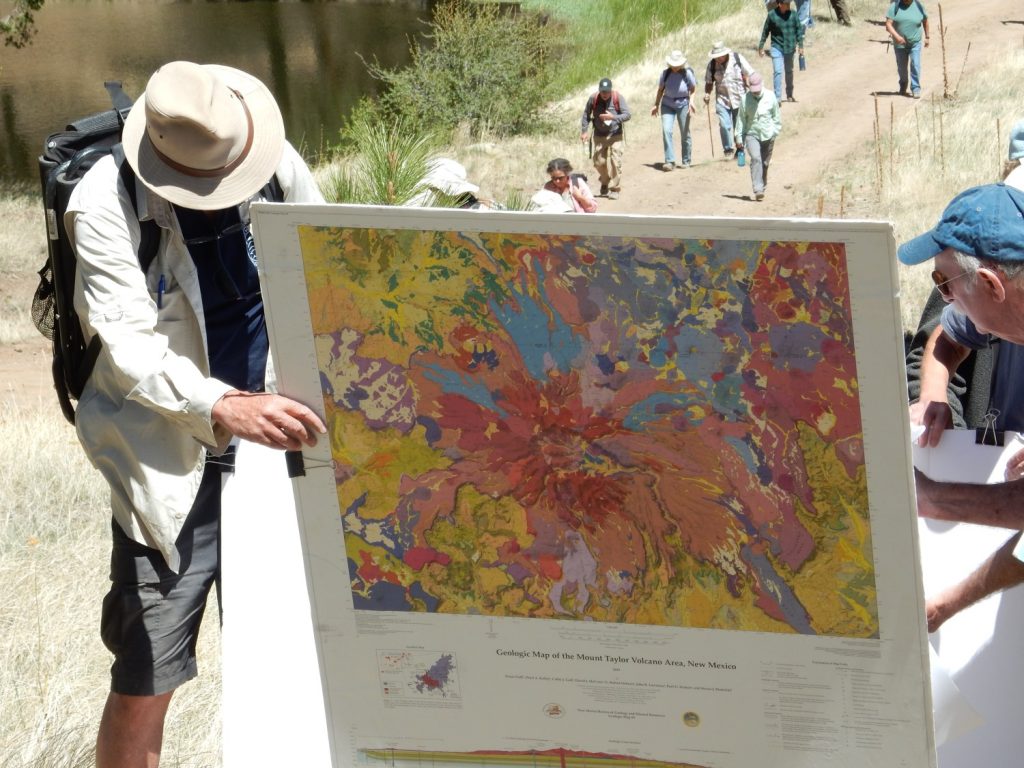
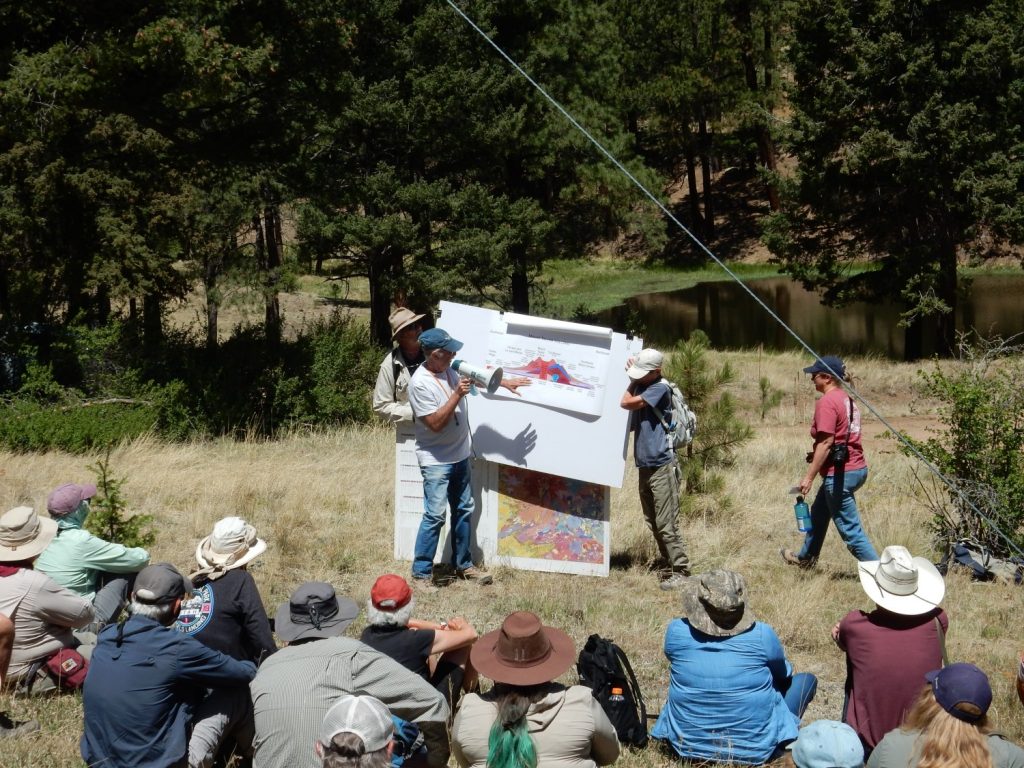
Then we troop off to see other interesting features of the Amphitheater:
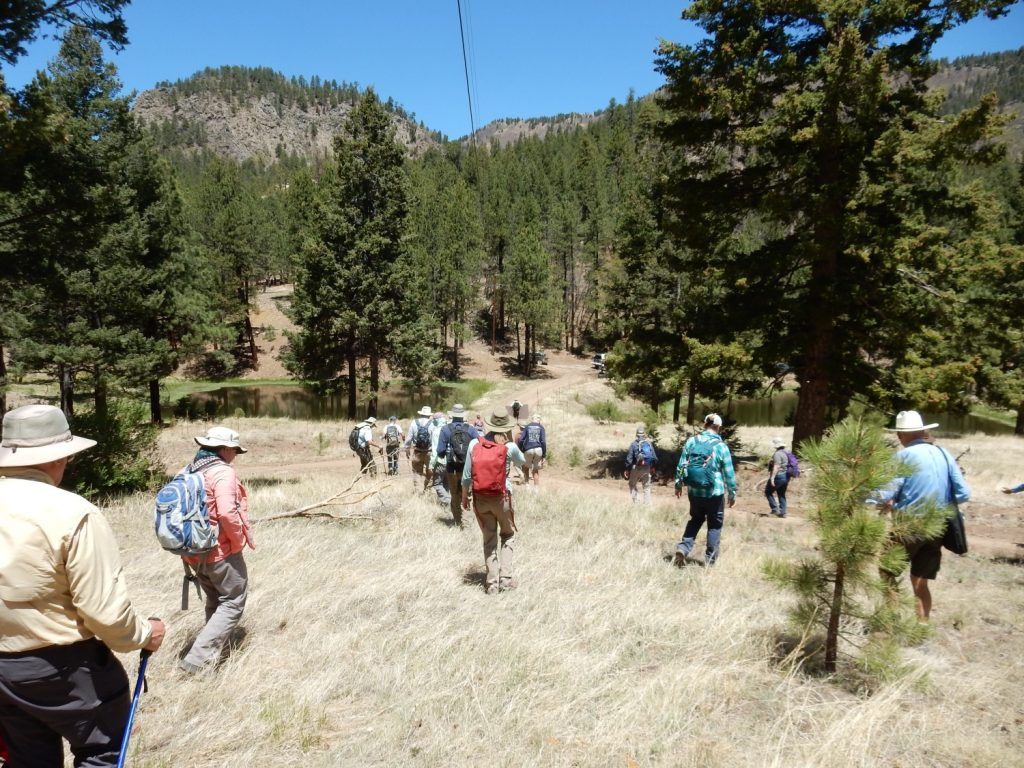
This is a thick dike of hornblende-biotite trachydacite, which (unsurprisingly) contains phenocrysts of hornblende and biotite. No collecting here, alas. This dike has been dated at about 2.64 million years old, making it part of the third phase of volcanism.
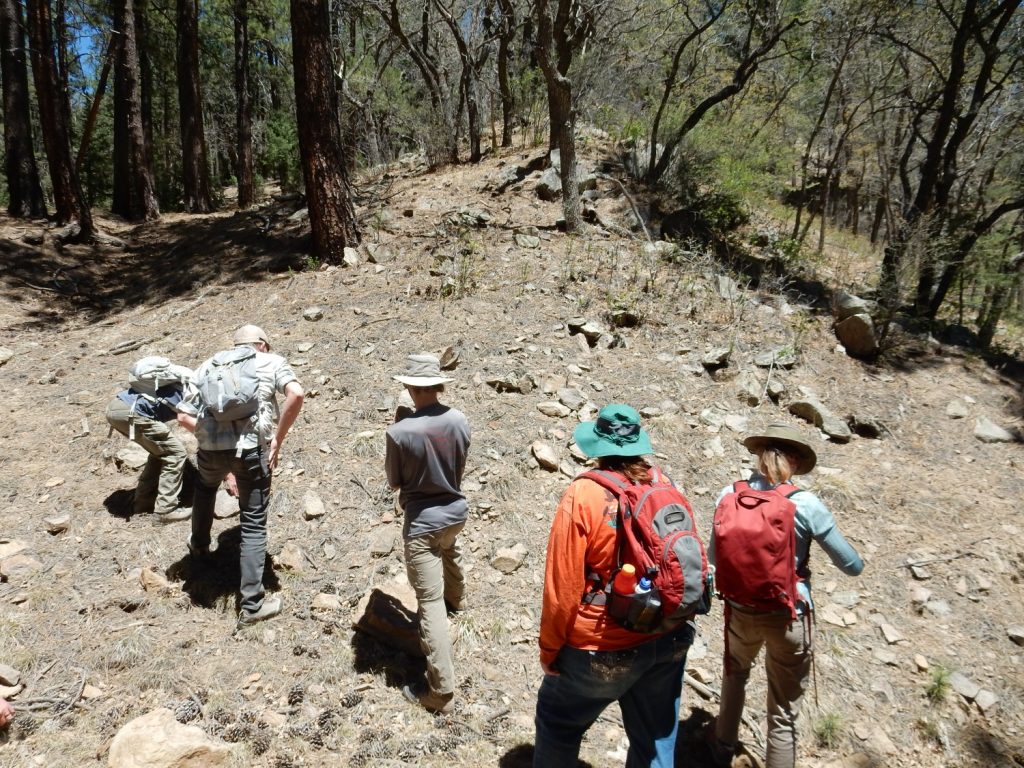
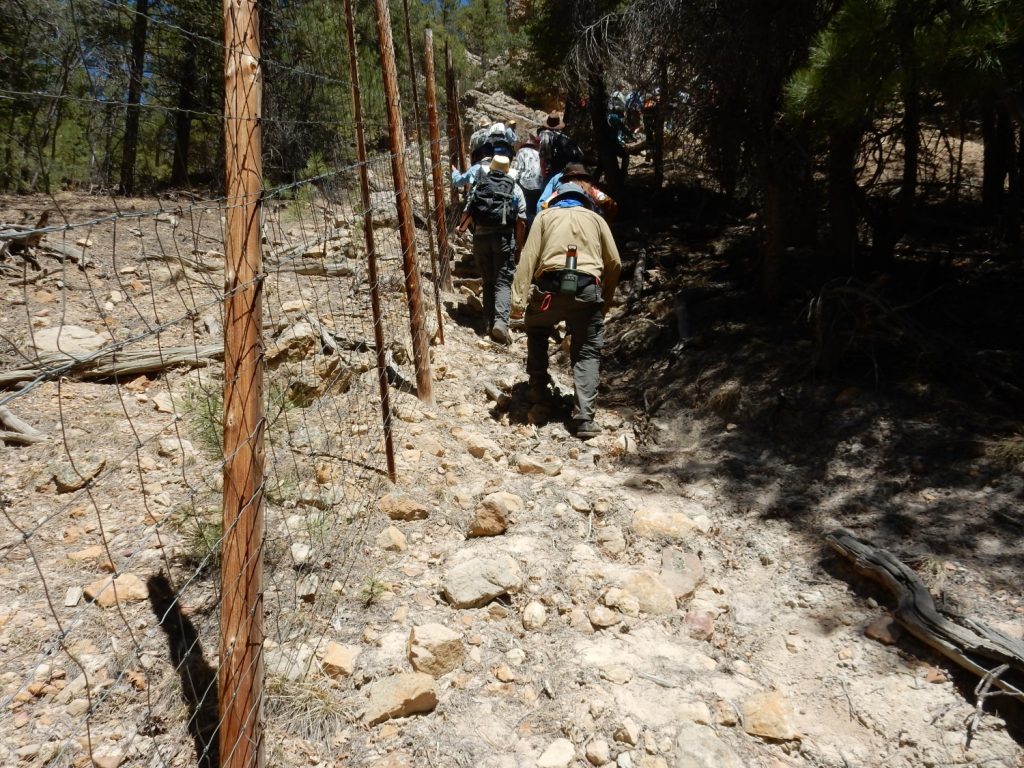
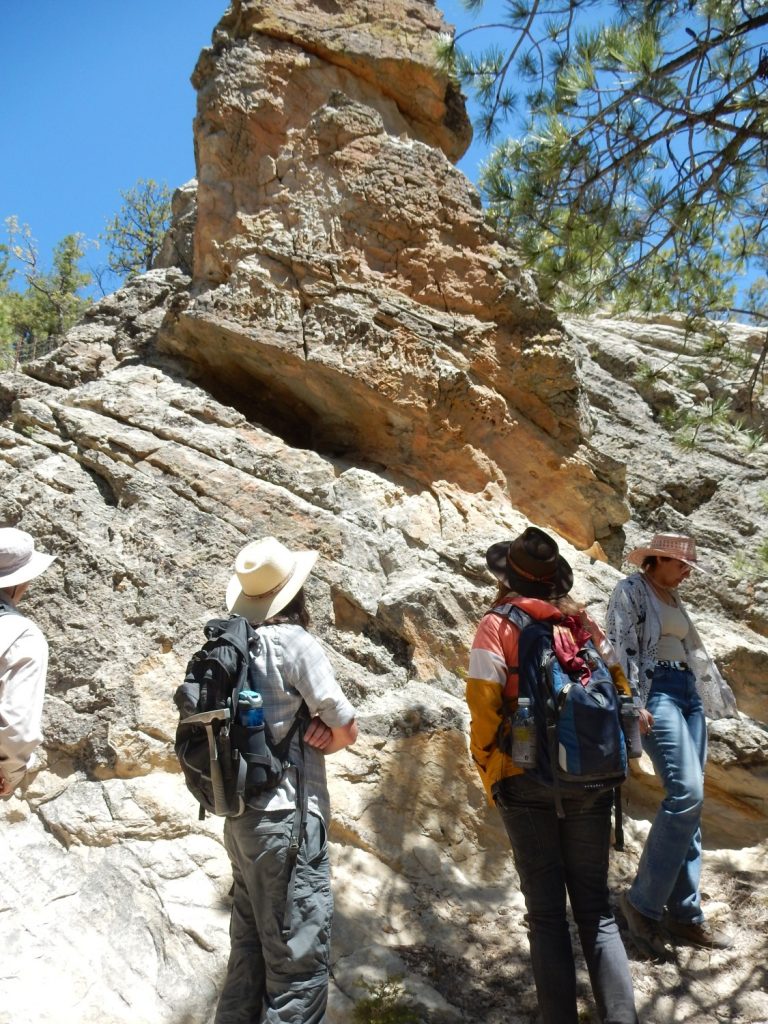
We are looking at somewhat cooked beds of Cretaceous sandstone under the floor of the volcano, exposed by erosion. Their presence helps rule out the possibility that the Amphitheater is a collapse caldera. These beds have been tentatively identified as Hosta Tongue of the Point Lookout Sandstone. We’re here to look for clues to firm up (or challenge!) that identification.
“Tongue”: This is a bed of one formation that intrudes laterally between beds of another formation. Intertonguing takes place when the boundary between two depositional environments moves back and forth. For example, the Hosta Tongue likely is beach sand, deposited between deeper marine shale beds as a shoreline briefly retreated.
Note the steep dip of the beds. The gabbro dome was intruded just to the west and tilted these beds.
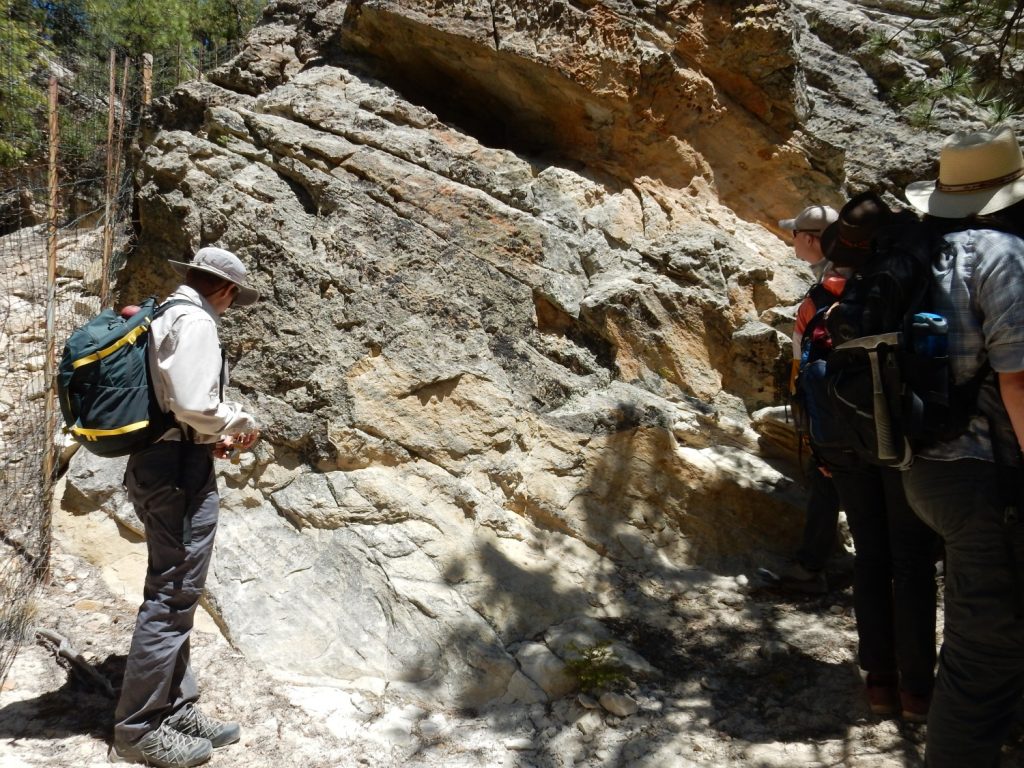
It looks like Point Lookout Sandstone under the loupe, but a lot of Cretaceous sandstones look like Point Lookout Sandstone under the loupe.
Sedimentary structures.
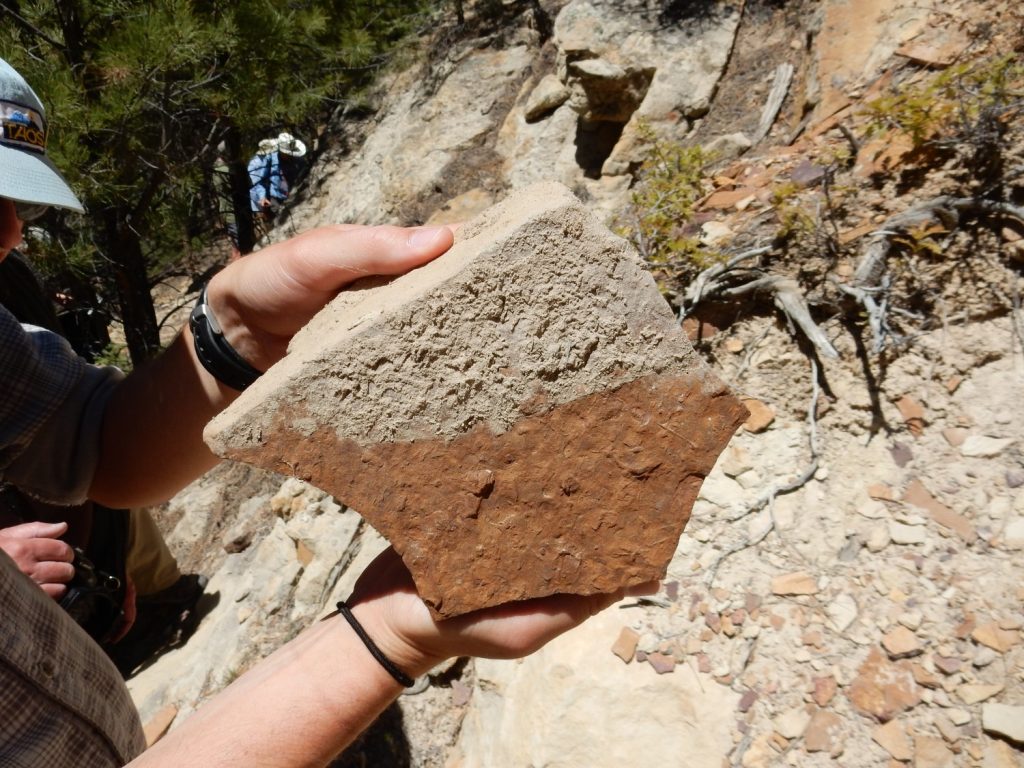
Concretions and a likely worm tube.
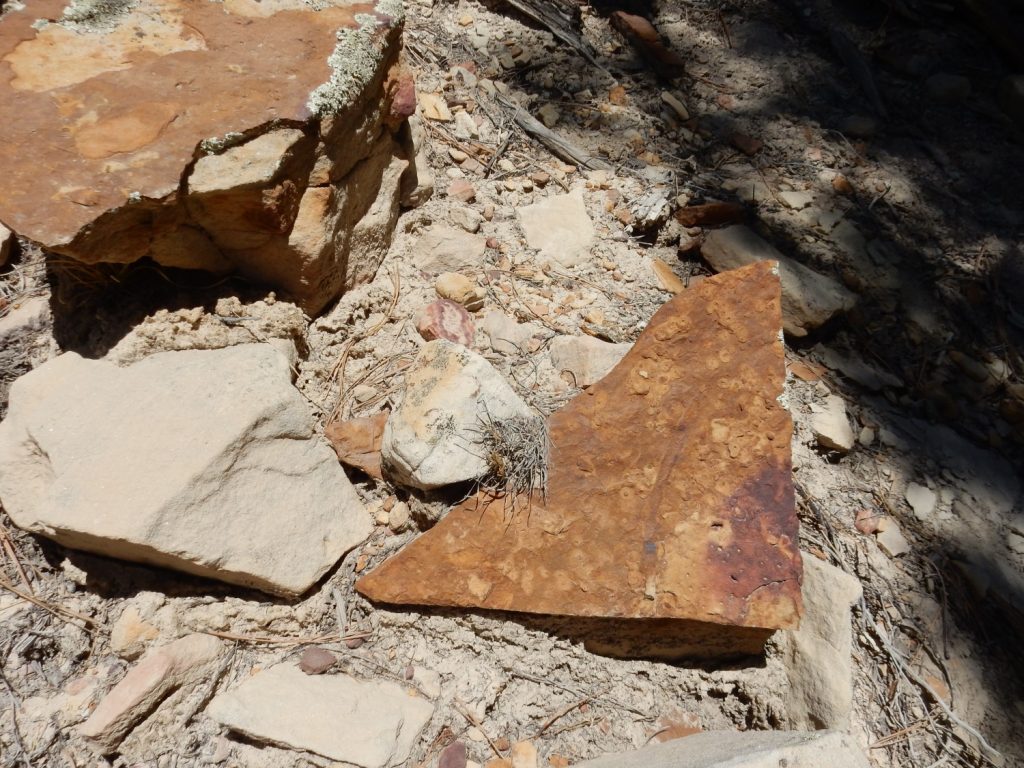
Worm tube or wood imprint?
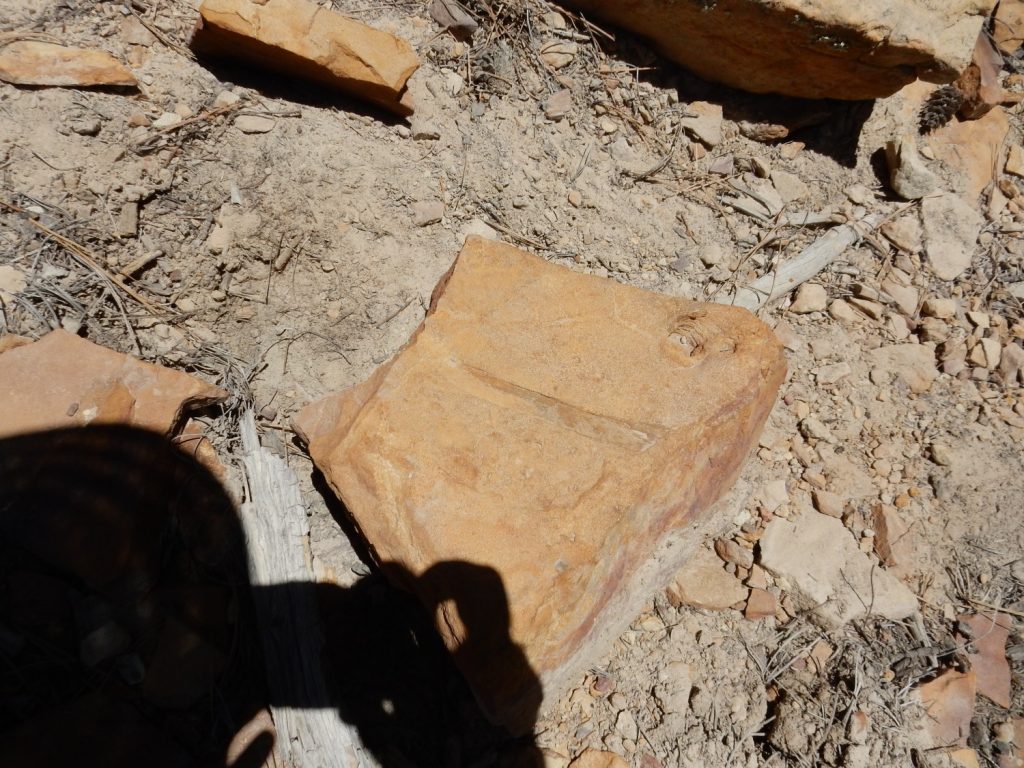
Sedimentary structures or trace fossils.
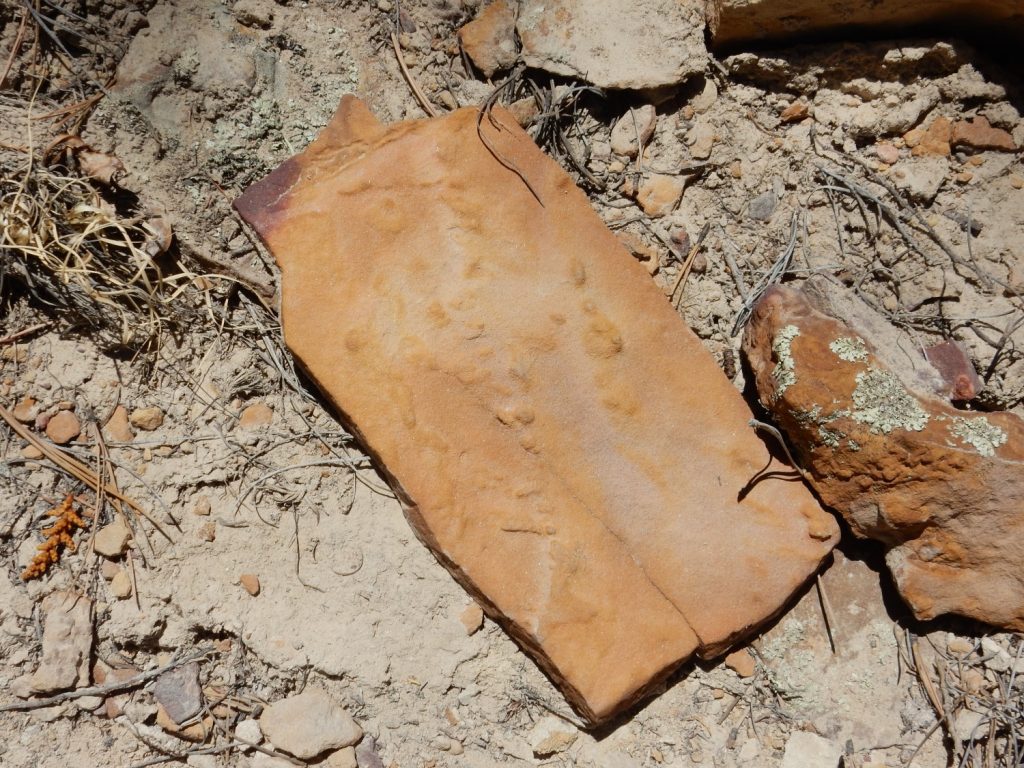
Definite fossil traces.
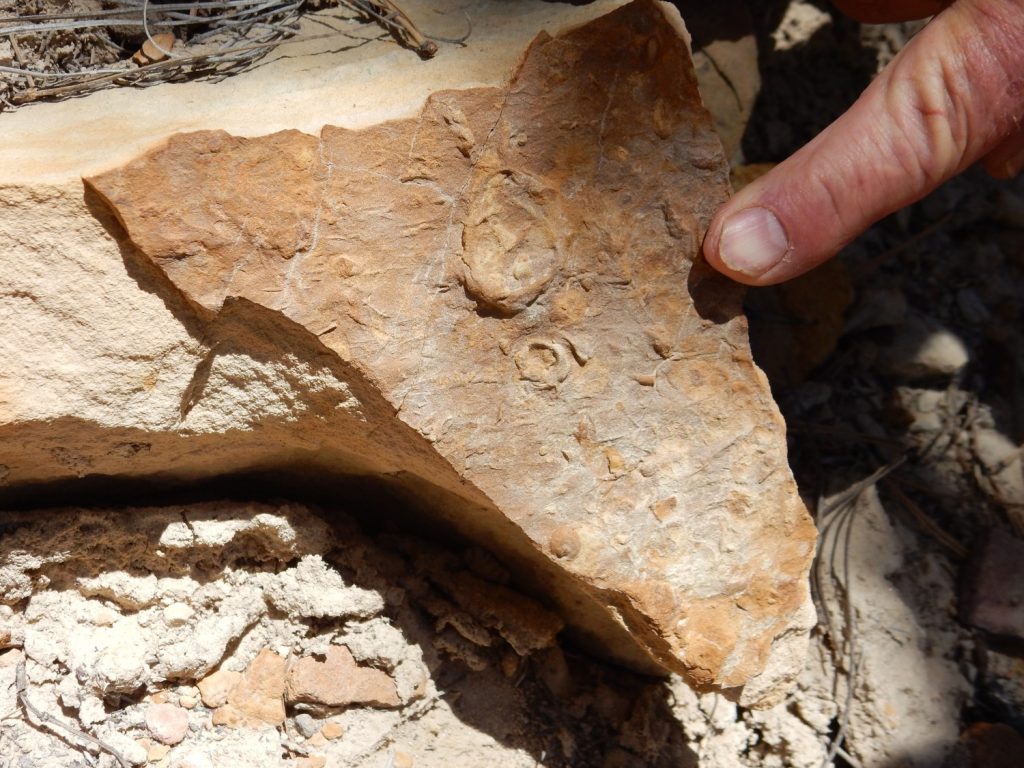
This looks a little like the trace fossil Thalassinoides.
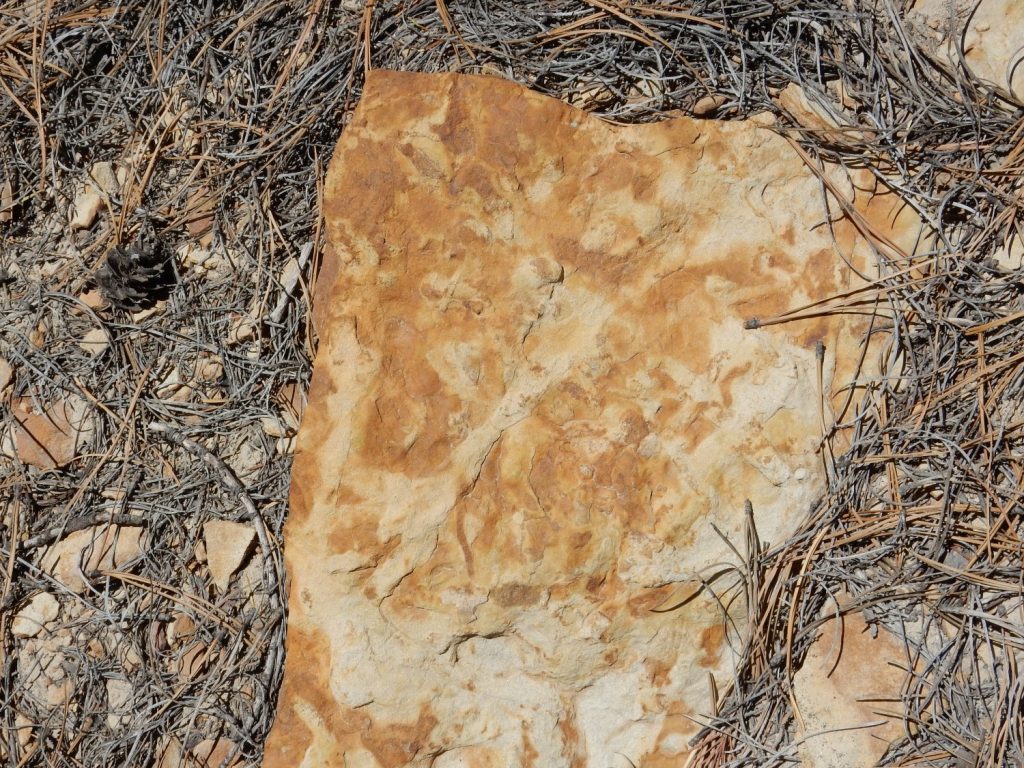
Thalassinoides are burrows produced by a number of organisms, but most commonly crayfish or their relatives.
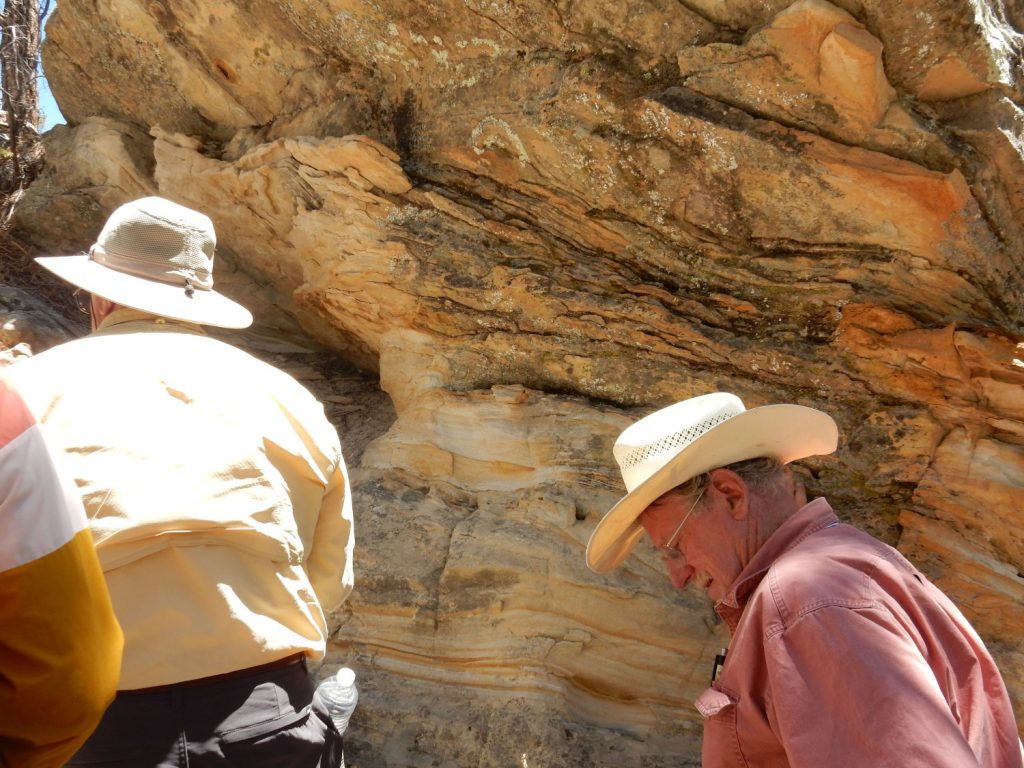
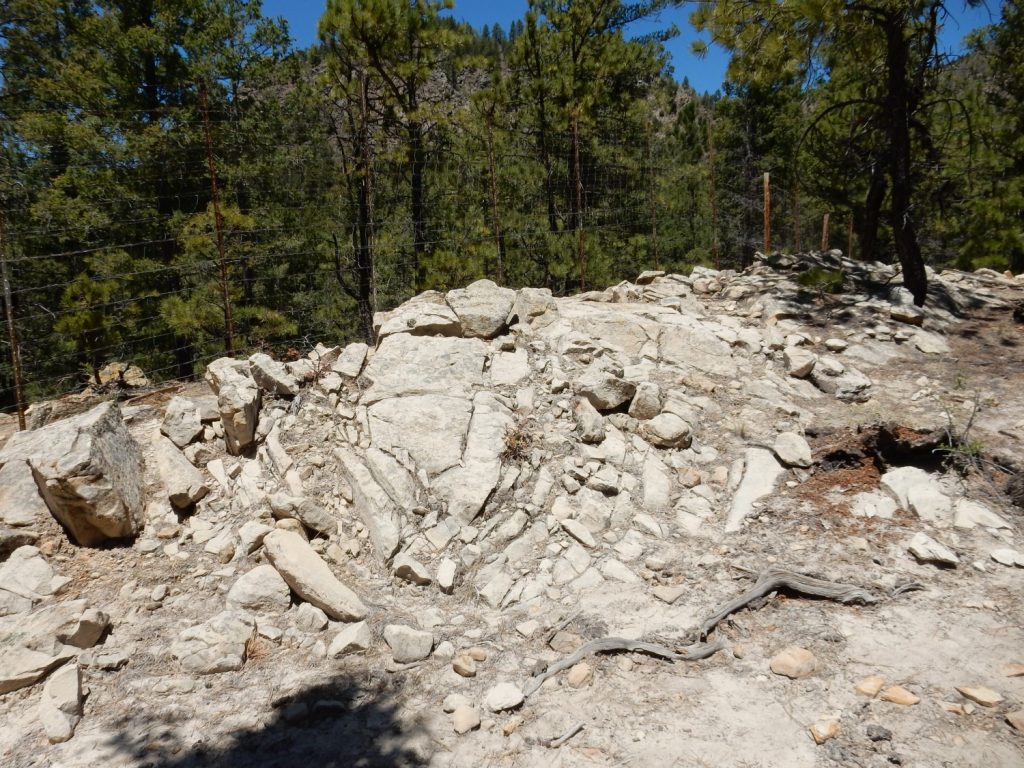
Unfortunately, nothing we’ve seen is age-diagnostic; that is, distinctive enough to help constrain the ages of these beds.
Nice view from here to the west.
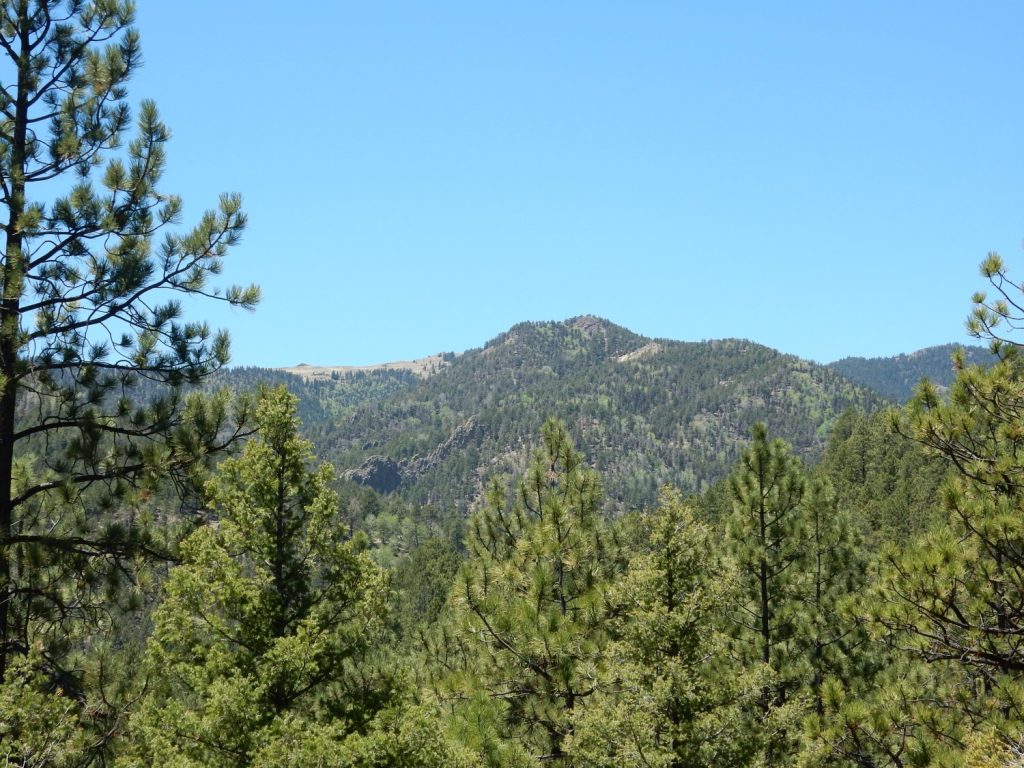
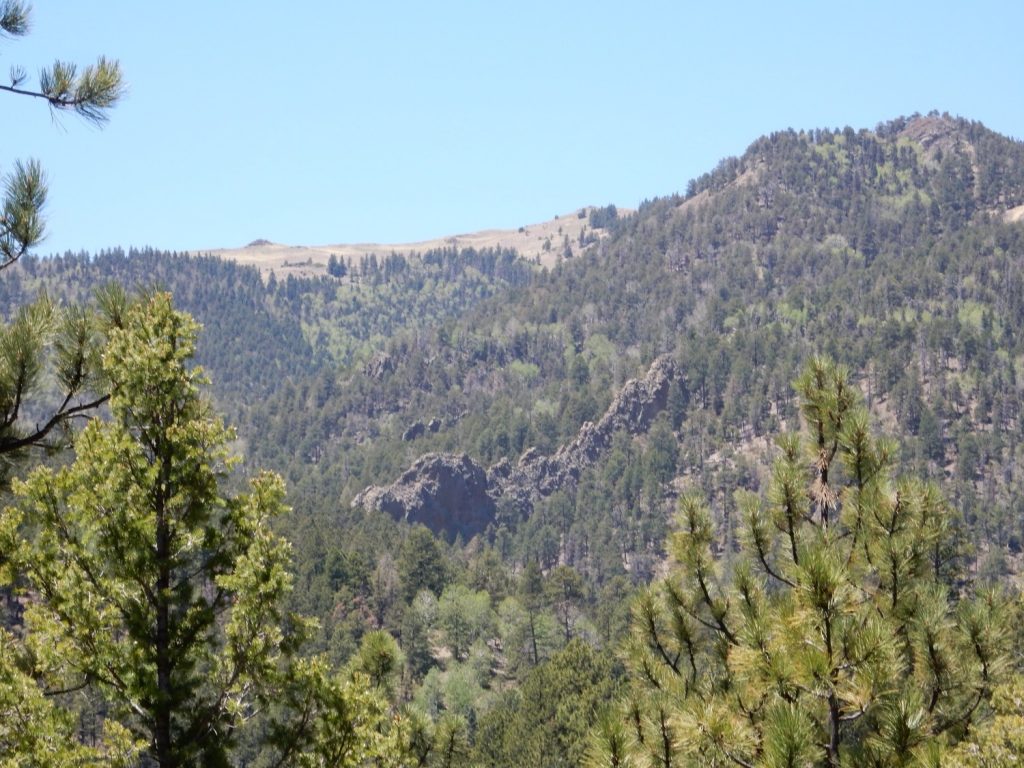
We are looking here at the central stock of the caldera, a mass of intrusive rock about 2.56 million years old, composed of trachydacite to alkaline rhyolite. This was the main feature of the third phase of volcanism. The dike is one of several dikes radiating from the stock, and it is about 2.77 million years old. This showed it formed early, before the main stock was intruded. The grassy rim beyond is the western rim of the amphitheater, some distance south of Mount Taylor peak itself.
Time for lunch.
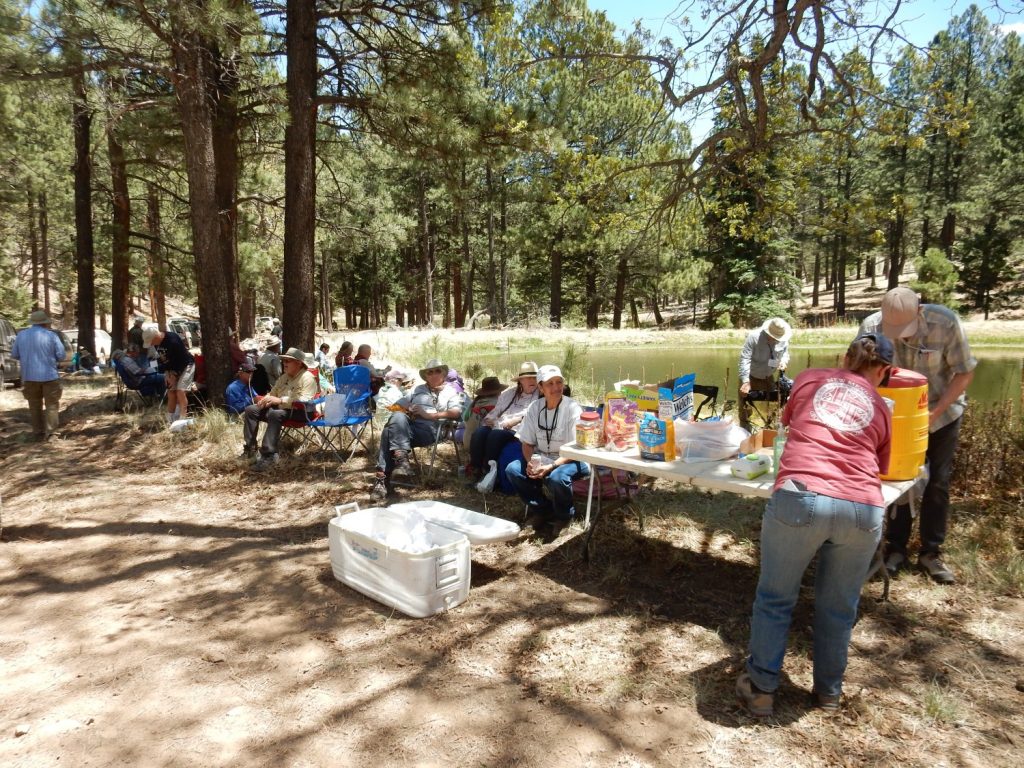
Another plug southeast of the area.
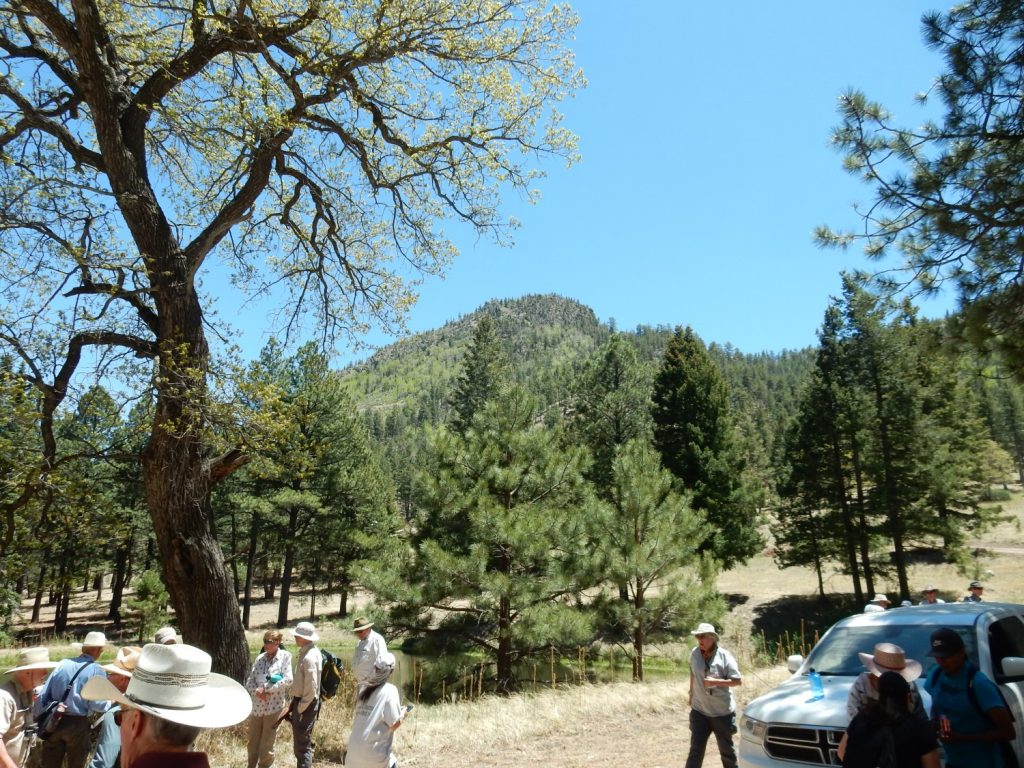
This is described on the map as a dome-and-flow complex, a satellite to the main stock of the Amphitheater, composed of coarse porphyritic trachydacite 2.7 million years old.
We troop off to see a couple more features.
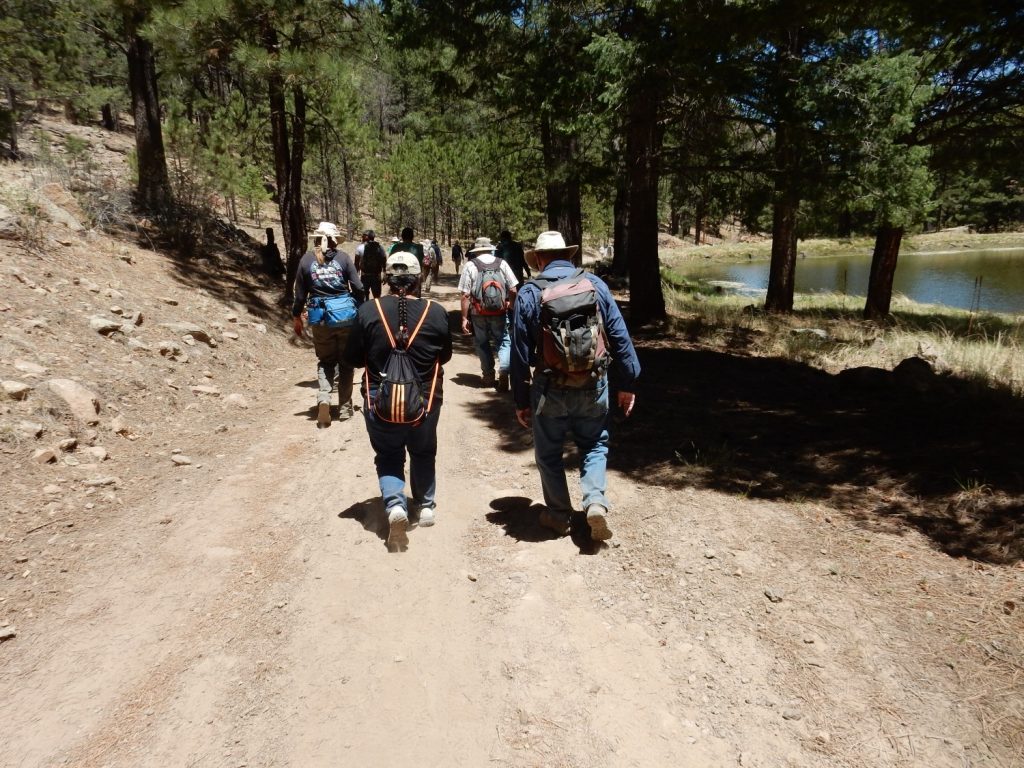
This outcrop looks like hornfelsized shale.
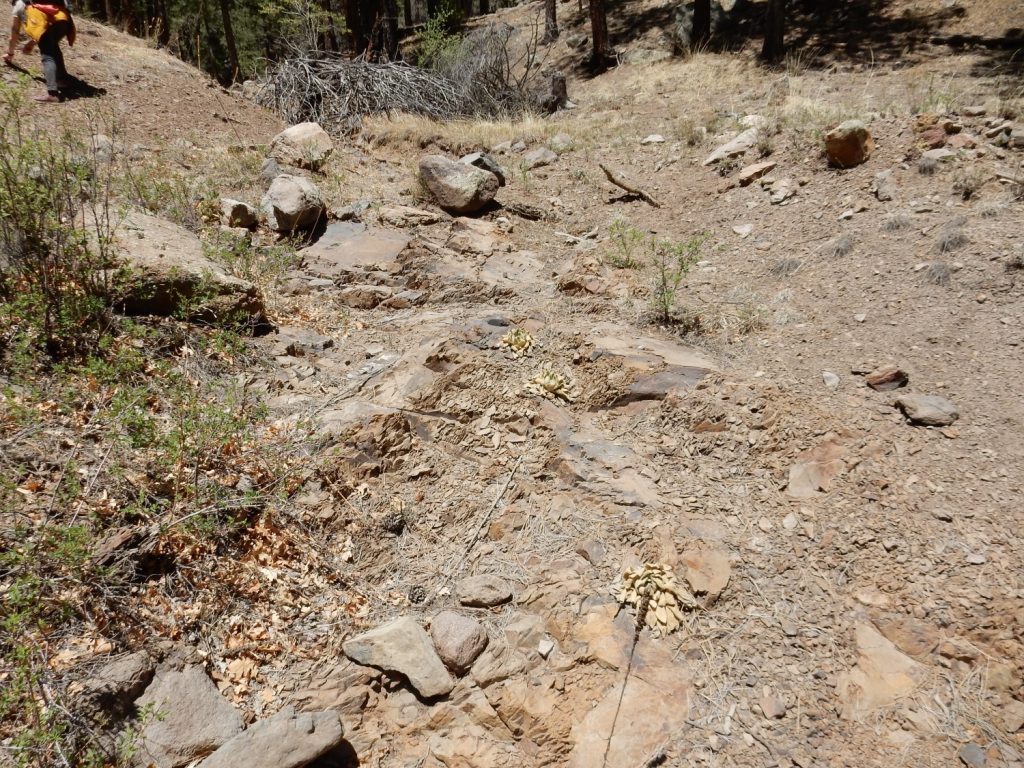
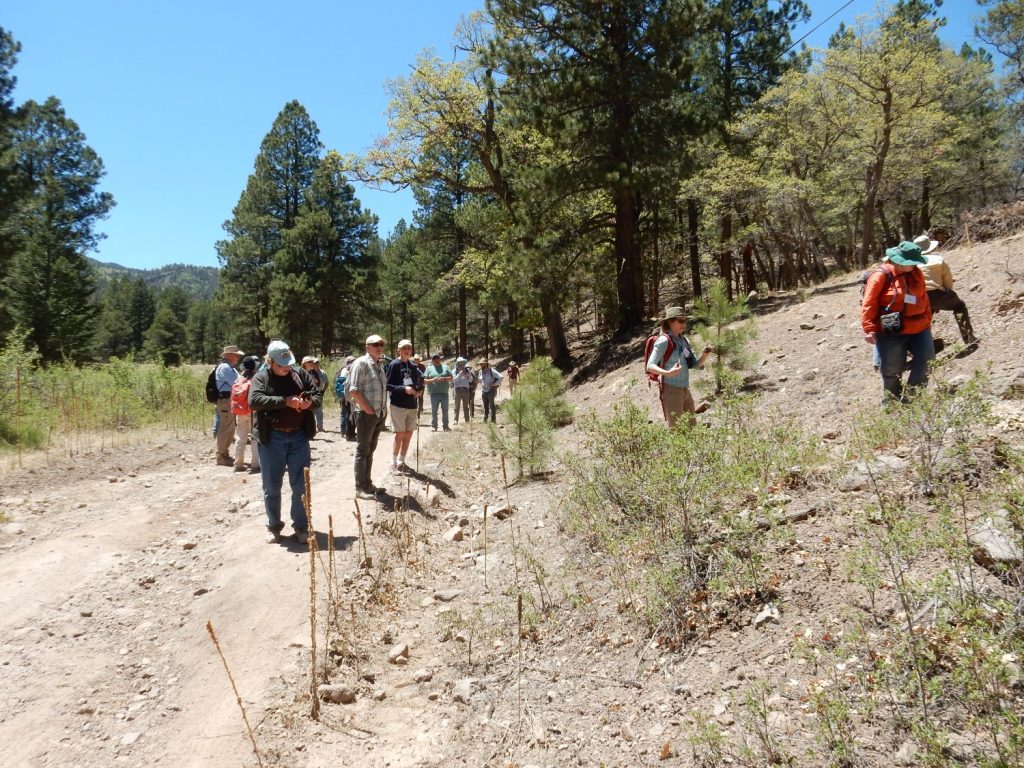
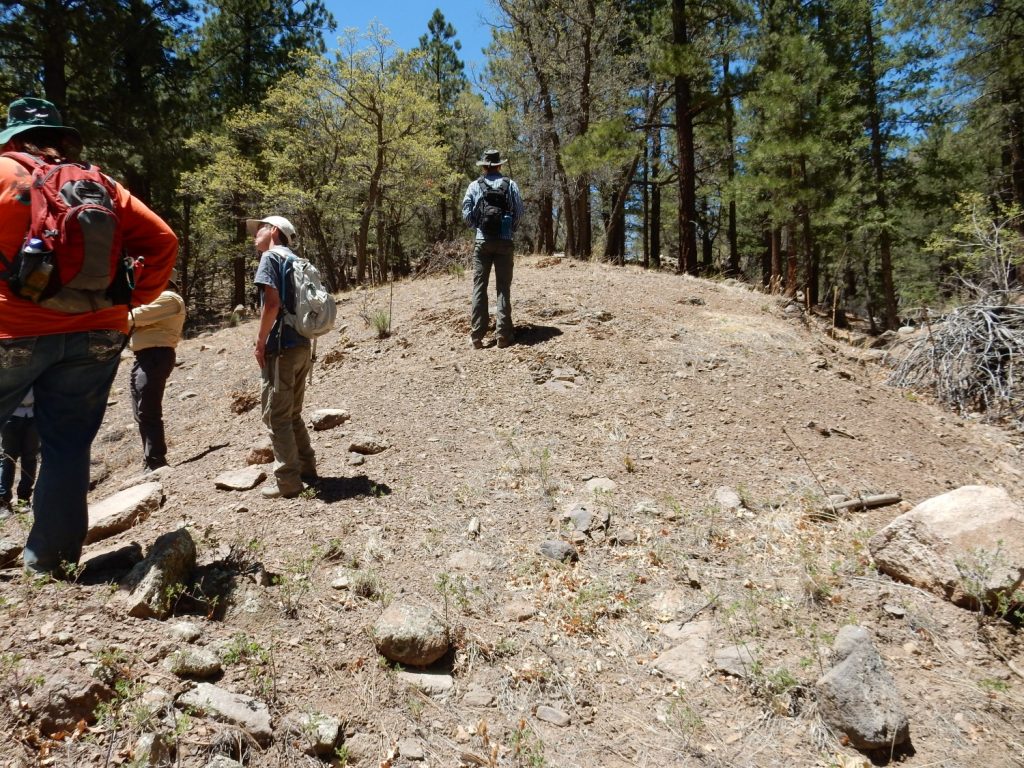
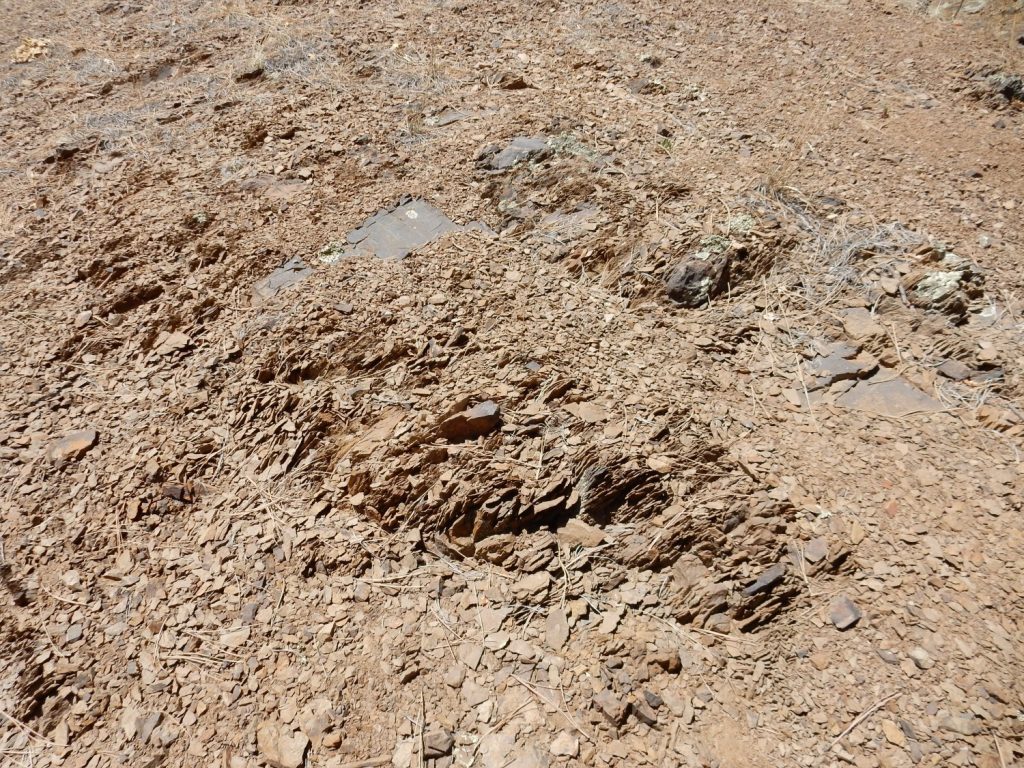
This has been identified as the Satan Tongue of the Mancos Shale. Here, in the bowels of a volcano, that seems somehow fitting. (This is not, however, how the Satan Tongue got its name. It is named for Satan Pass, well to the northwest.) The shale, normally a very soft, flaky sedimentary rock, has here been cooked into hornfels, a much harder rock in which much of the flaky structure has been obliterated.
The posters come out for a discussion of Mount Taylor tuffs.
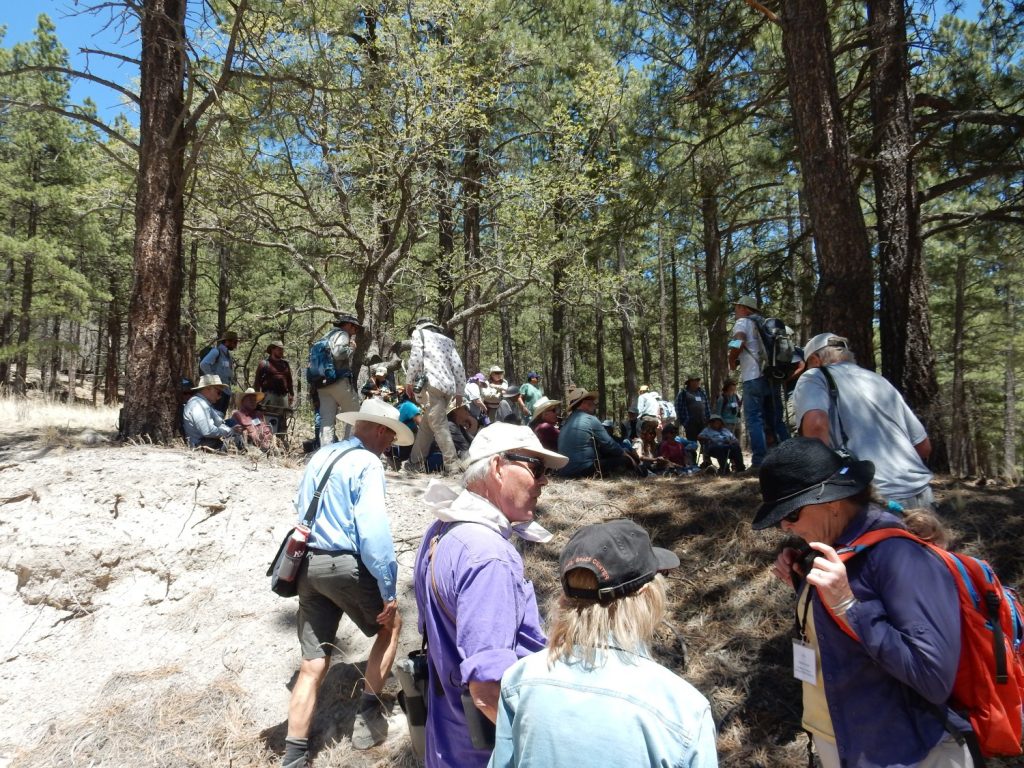
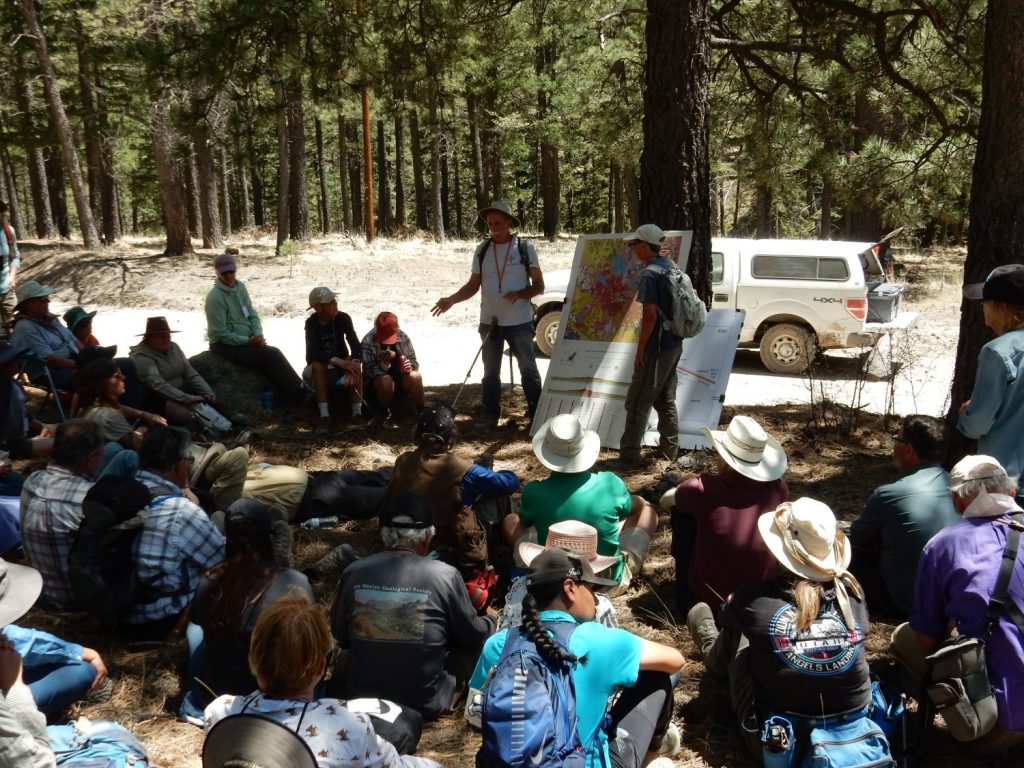
We will be looking at the tuff of Water Canyon up slope of this point. Off we go:
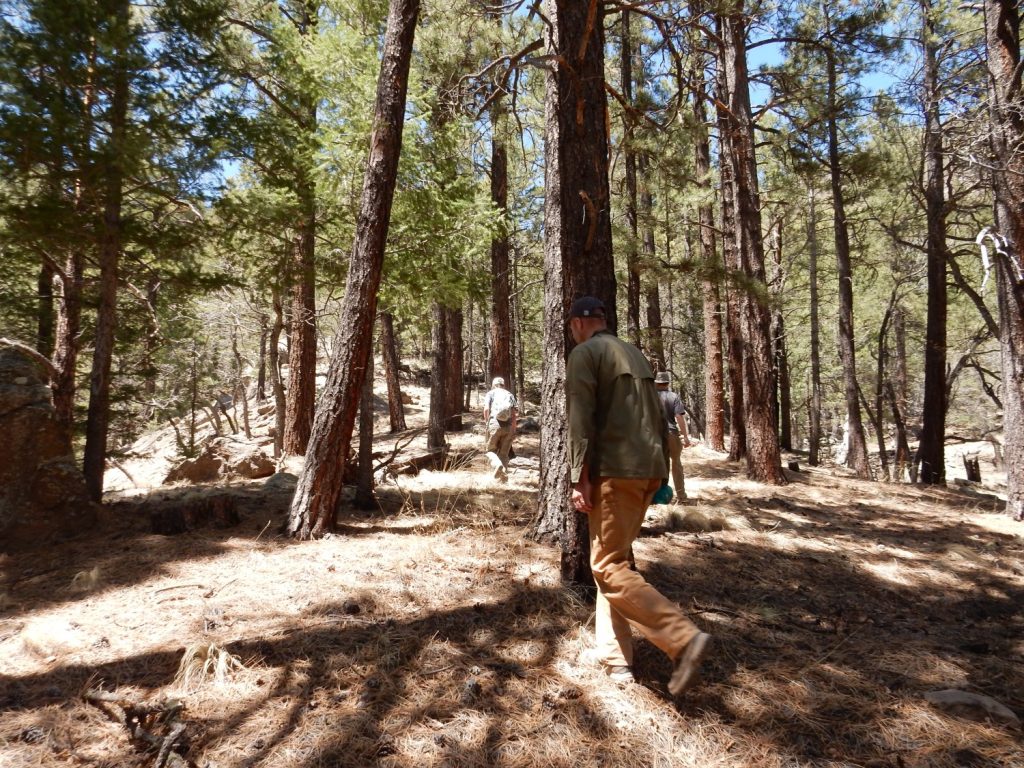
We reach the lower bed of the tuff.
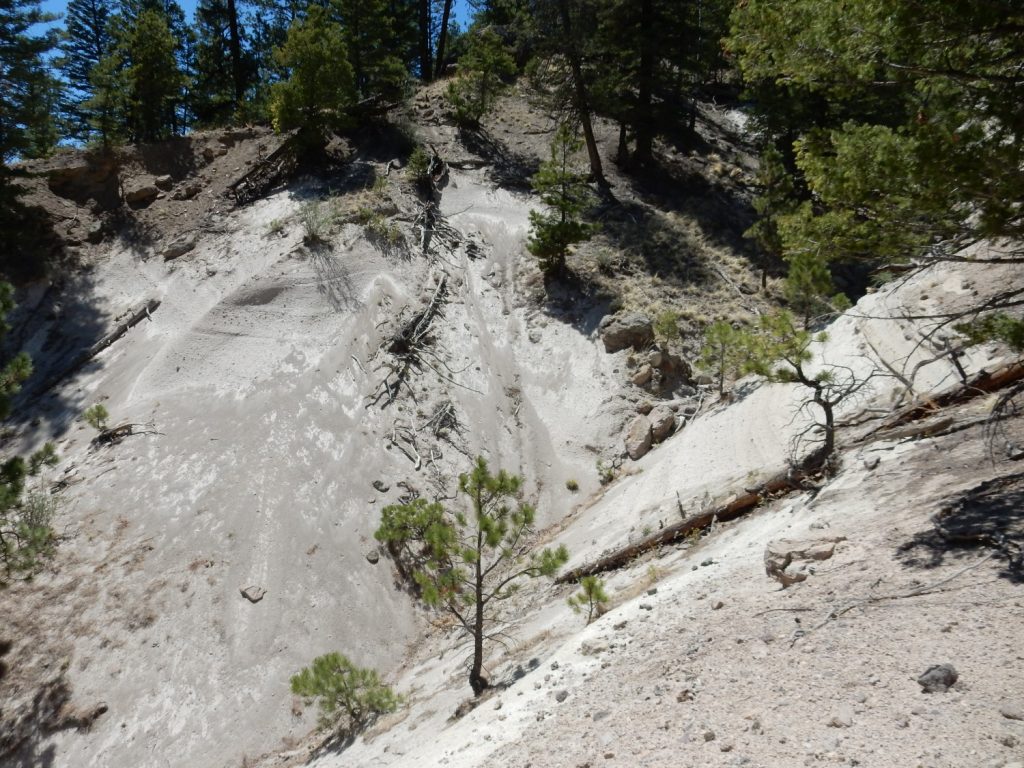
This is a massive, nearly featureless tuff, with few lithic fragments, and likely unwelded. Let’s unpack that: This is a bed of volcanic ash, which flowed out across the surface as a pyroclastic flow — a mixture of tiny volcanic glass fragments and red-hot gas that flowed like a heavy liquid. The ash settled to the surface and cooled to become tuff. This was likely erupted in one massive belch, with perhaps some very slight pauses towards the end producing the weak bedding we see across the way. There were very few solid rock fragments (lithic fragments) in this flow; some tuffs are rich with rock fragments, called lithic clasts, torn from the walls of the vents from which they erupted. There is also little indication that the ash was so hot when it settled onto the surface that it welded, with the glass fragments melding together into a very solid mass. But it takes petrographic analysis in a laboratory to verify that. The tuff is harder than it looks, but one of the geologists (either one of the Kelleys or Fraser Goff; I don’t recall) mused that that was probably cementing by groundwater rather than welding.
Shari admires the exposure.
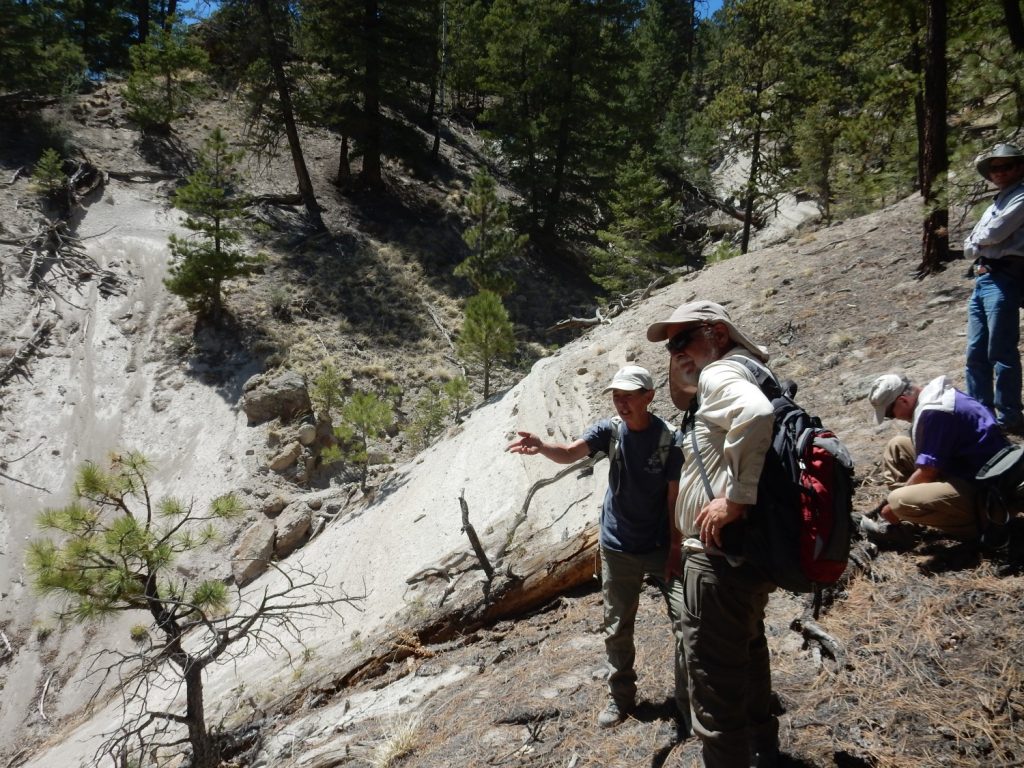
We continue up to beds that are more strongly cemented.
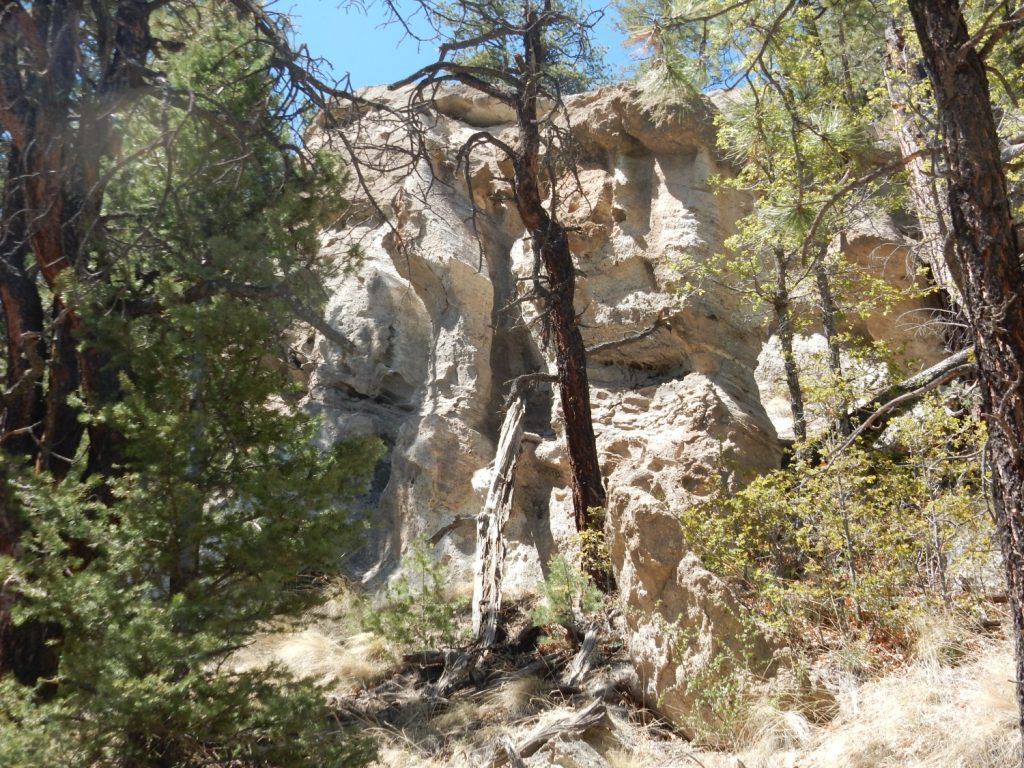
The exposures here show more bedding and other structure.
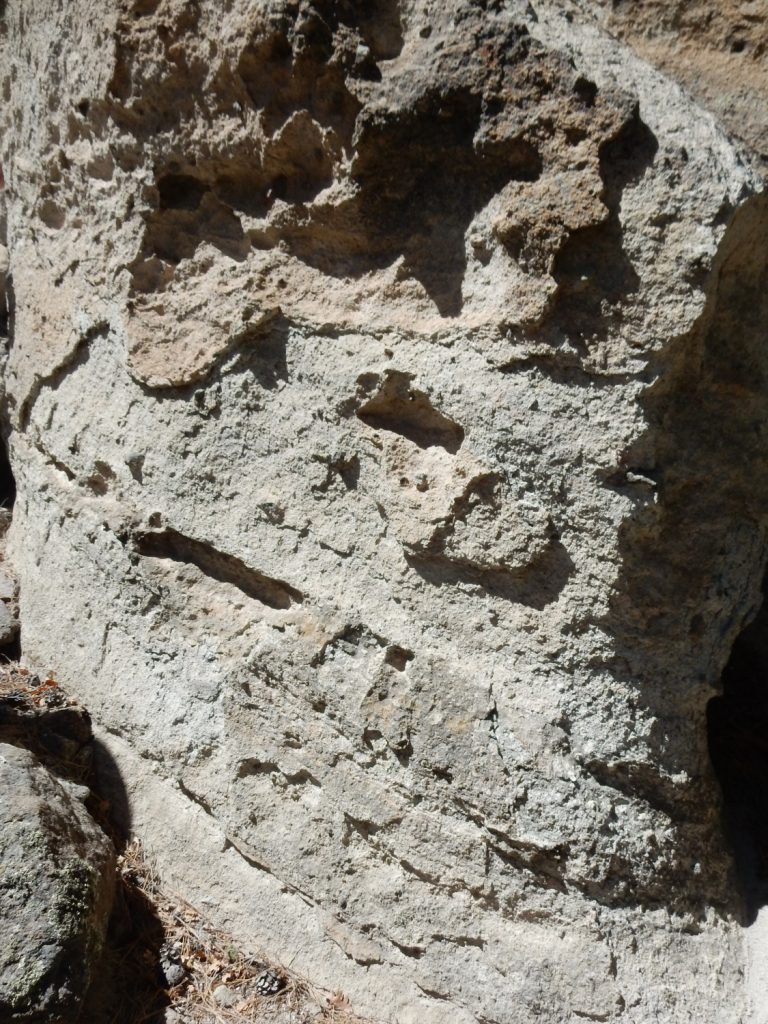
Sheri discusses some of the marker beds in the upper part of the tuff.
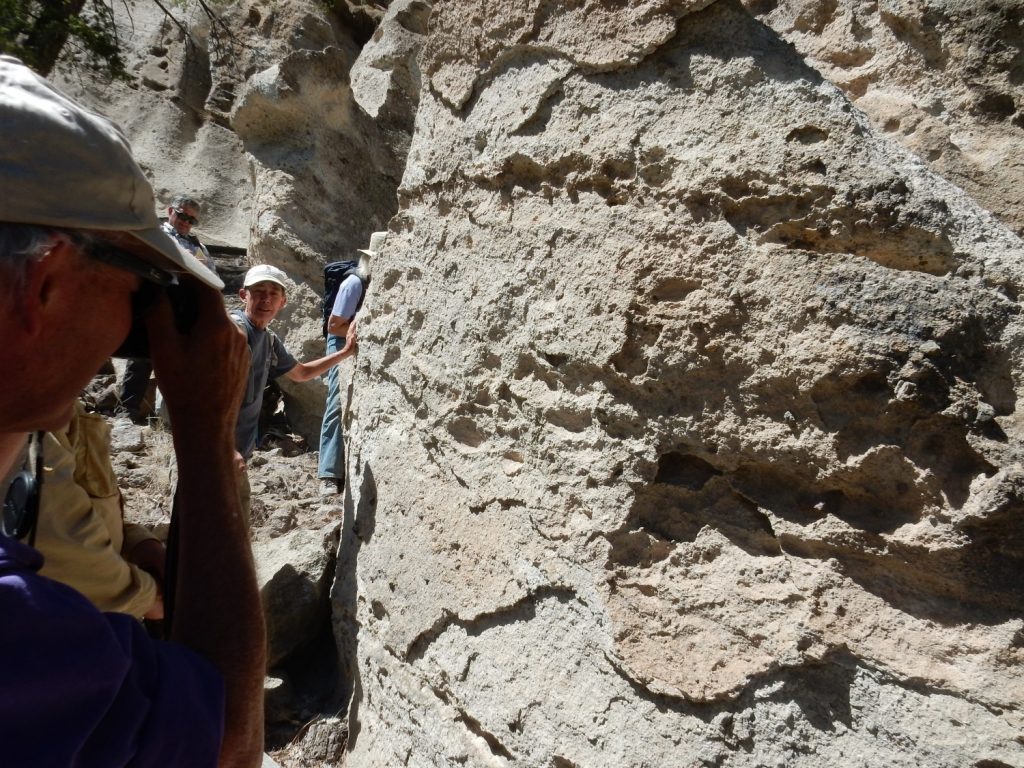
The colored bed here can be traced considerable distances.
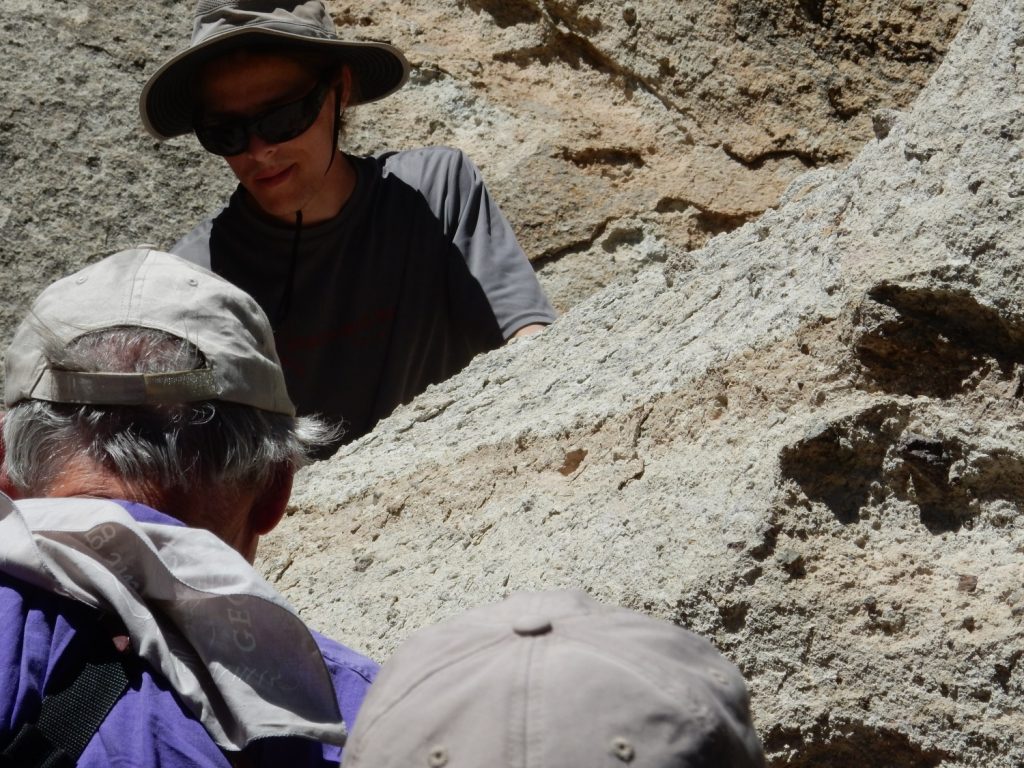
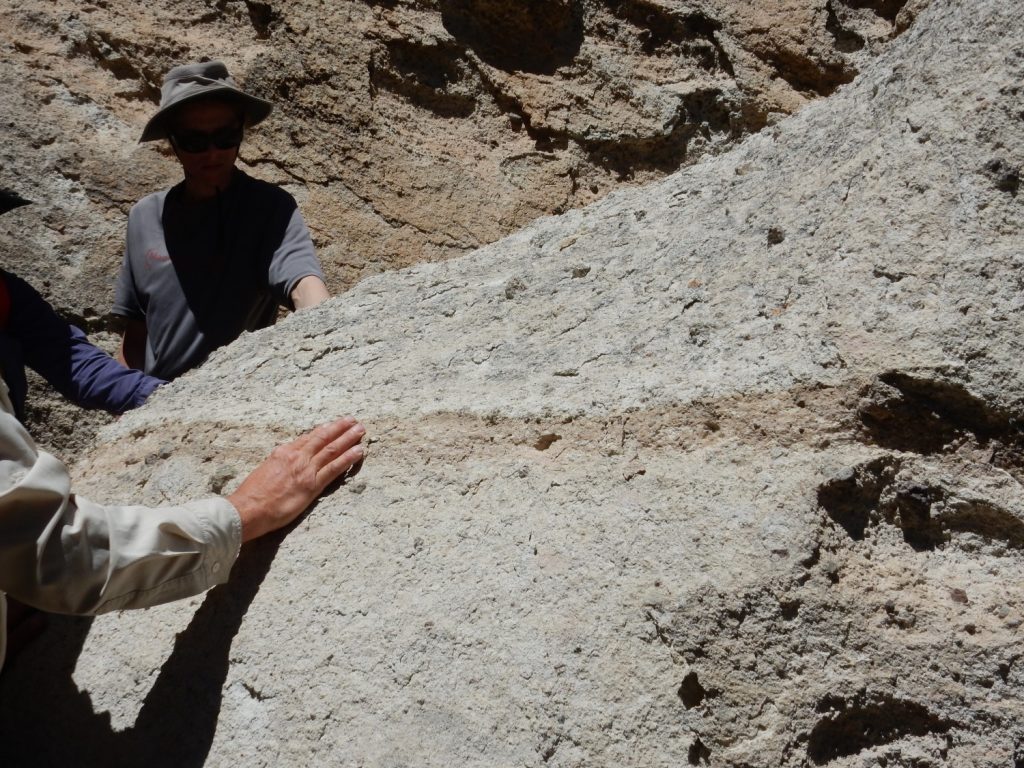
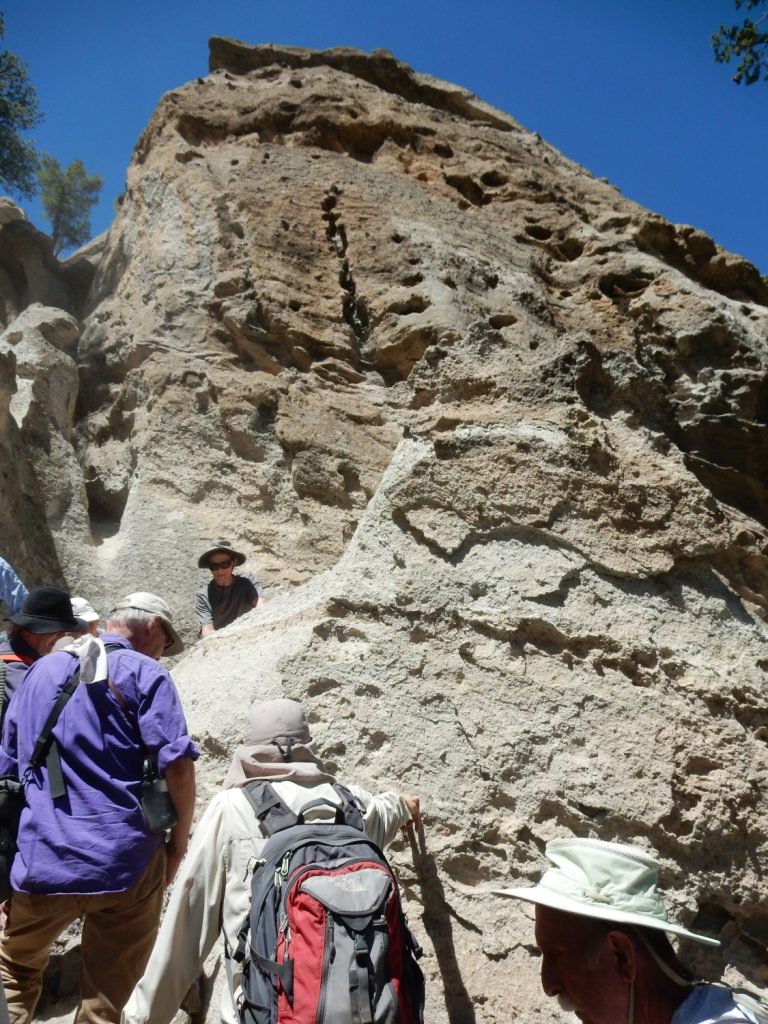
Further up is a distinctive set of surge beds.
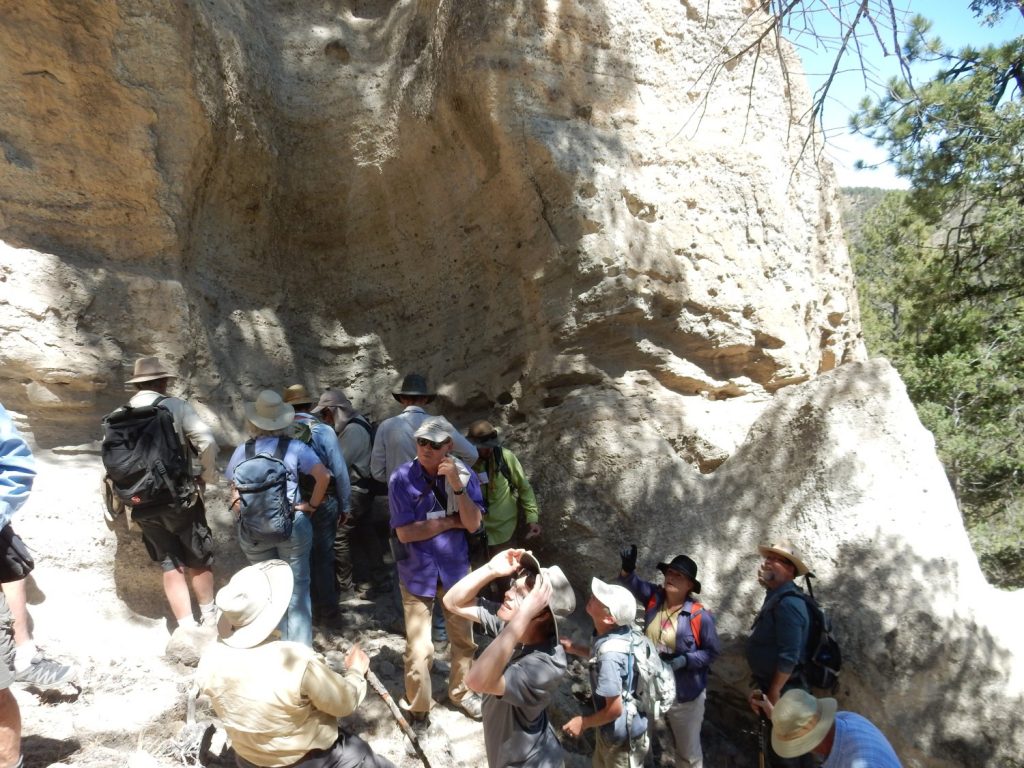
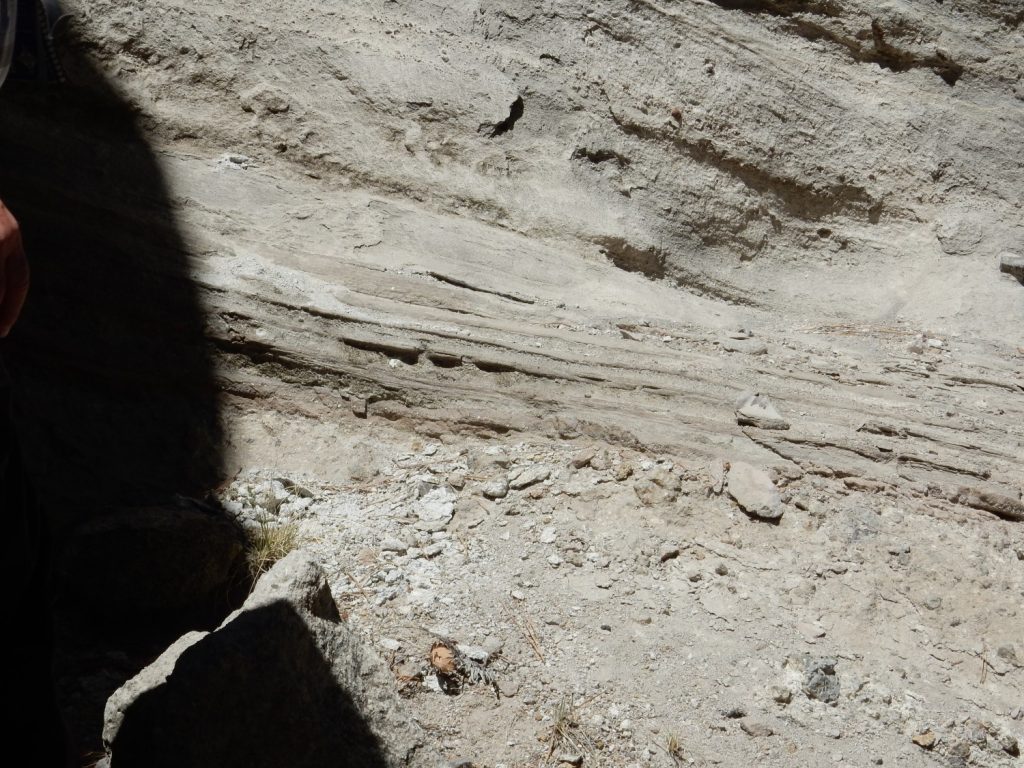
A pyroclastic surge is a bit like a pyroclastic flow, but hotter, less rich with volcanic ash, and more violent and deadly. Surges can flow short distances uphill, which pyroclastic flows rarely do, and they show sedimentary structure resembling sandstone deposited in a strong current. Here a pyroclastic surge left thin beds of ash. Pyroclastic surges tend to precede, follow, or be found to the sides of pyroclastic flows, and they move much faster, at hurricane velocities. It is now thought that it was a pyroclastic surge that killed nearly the entire population of St. Pierre on Martinique in 1902.
Above the surge beds is lithic-rich tuff.
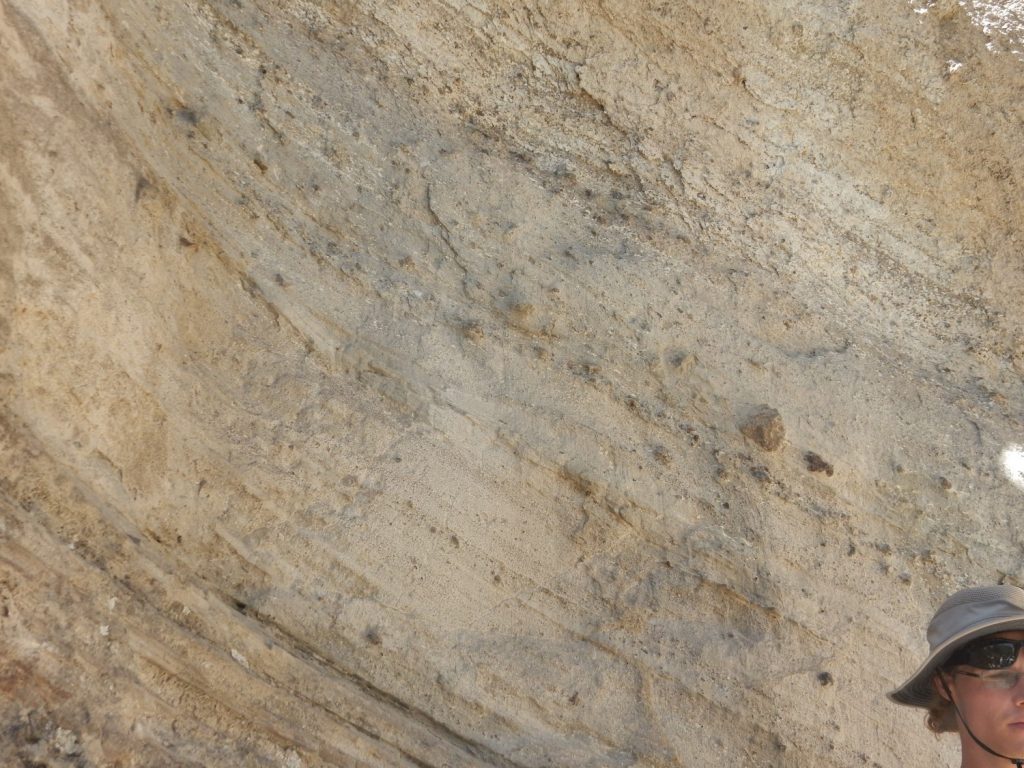
Look at the size of some of the rock fragments entrained in the pyroclastic flow.
Also note the steep dip on the beds to the east. This is similar to the dip of the Cretaceous beds we saw earlier, and suggests these beds were tilted by the same intrusion. It’s unlikely that a tuff would originally be laid down at an angle like this.
High overhead is a layer that is unusually well cemented.
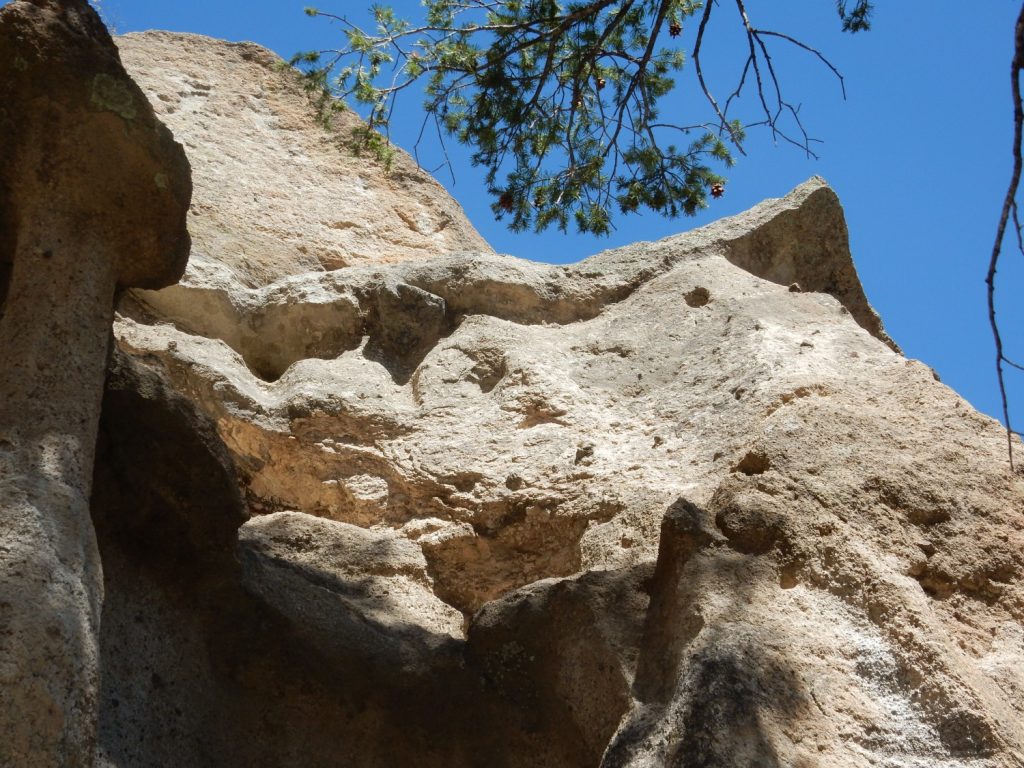
I don’t know what causes this. Not a surge bed; surge beds tend to be softer than massive tuff, sometimes forming notches in a set of beds.
Shari leads a few intrepid souls up higher to look at the very top of the tuff. Gary and I bow out; we are growing weary, and, besides, we do not want to miss the barbeque.
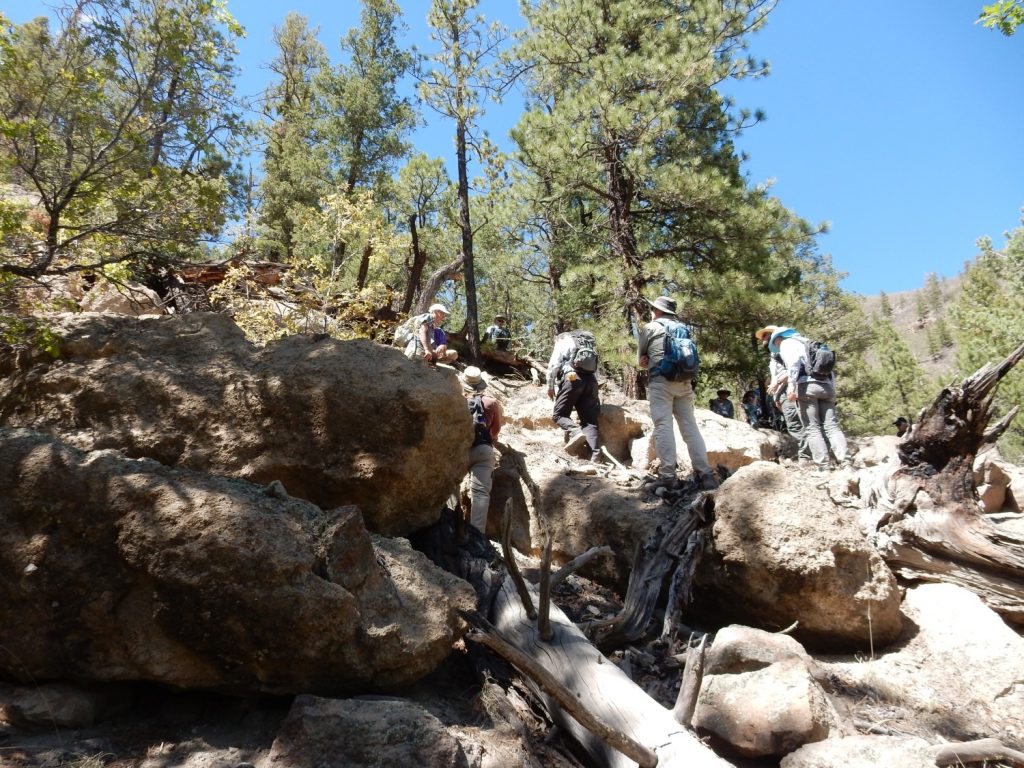
A final shot of tuff as we descend.
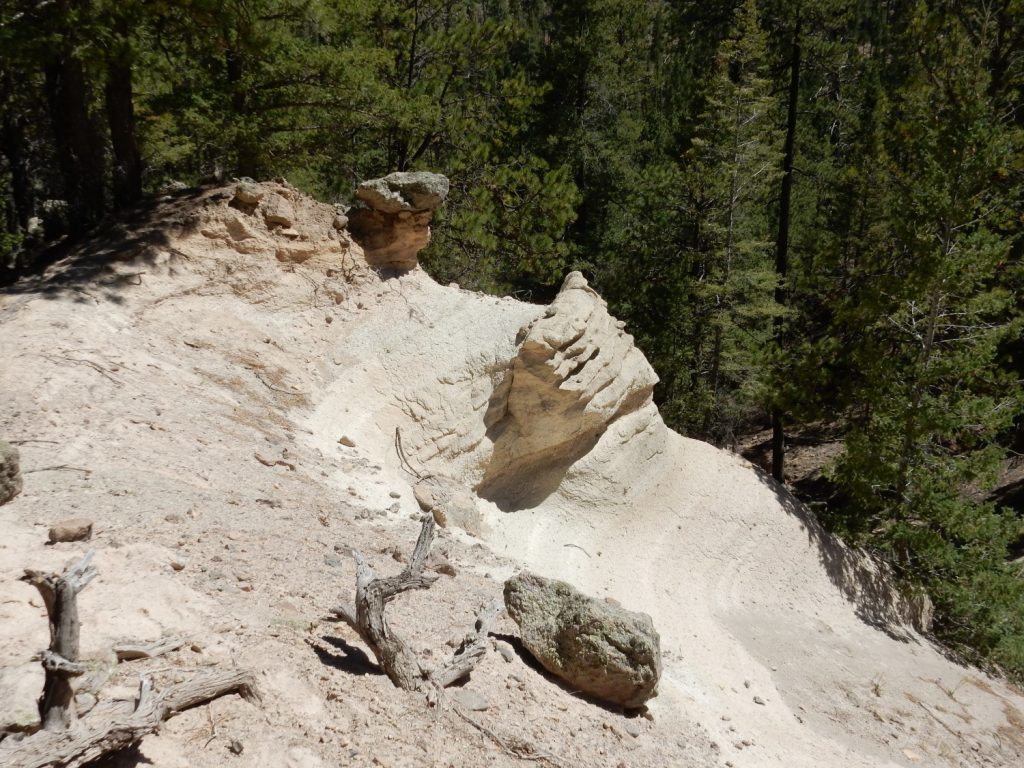
Then back down, into the cars, and off to Grants for the barbeque at the golf course.
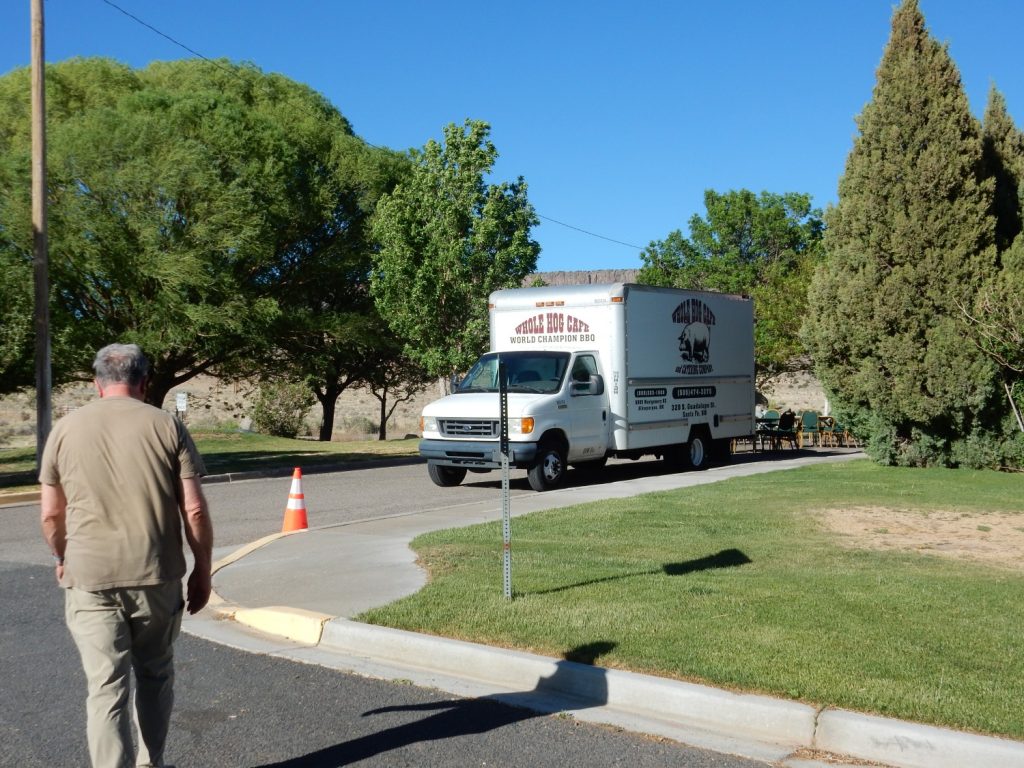
There is entertainment.
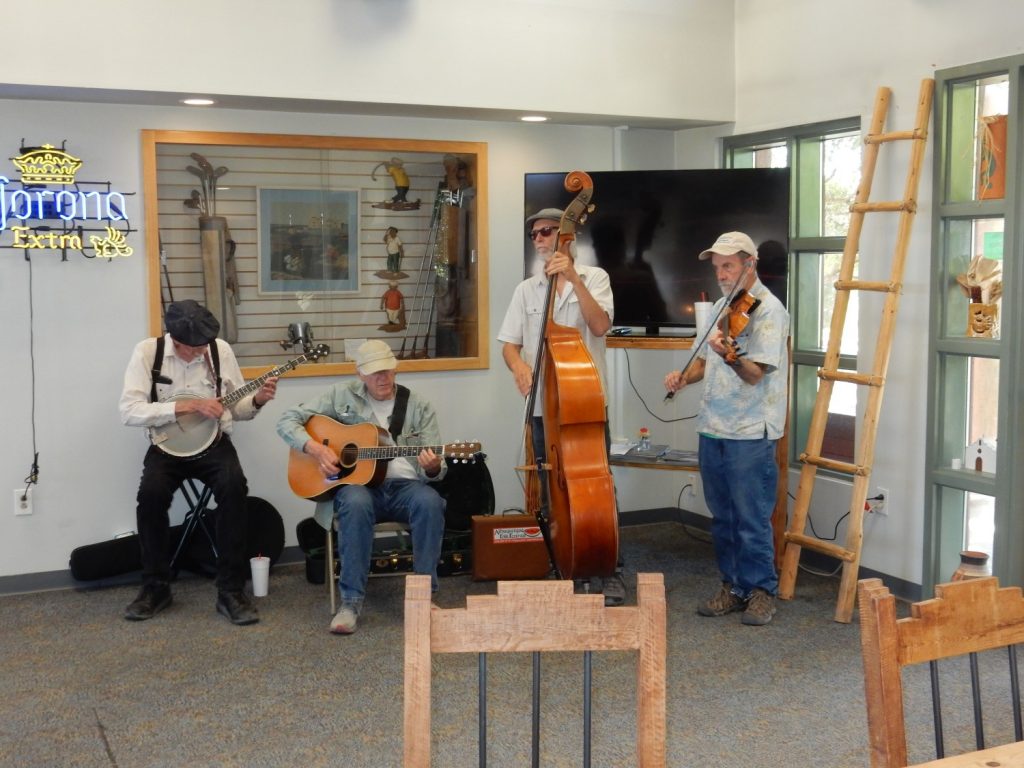
Gary Stradling and I have an interesting conversation with Nelia Dunbar and a couple of other geologists (James and Susan Cearley, possibly? Apologies; I’m not good with names) about the future of mining and of scientific education in New Mexico.
The barbeque is excellent. I will end up gaining a few pounds this trip, in spite of the exercise, which I am now working back off.
Then back to the hotel for the night.
Pingback: Mount Taylor Wanderlust, Day 6 | Wanderlusting the Jemez